Why Is The Plasma Membrane Called Selectively Permeable
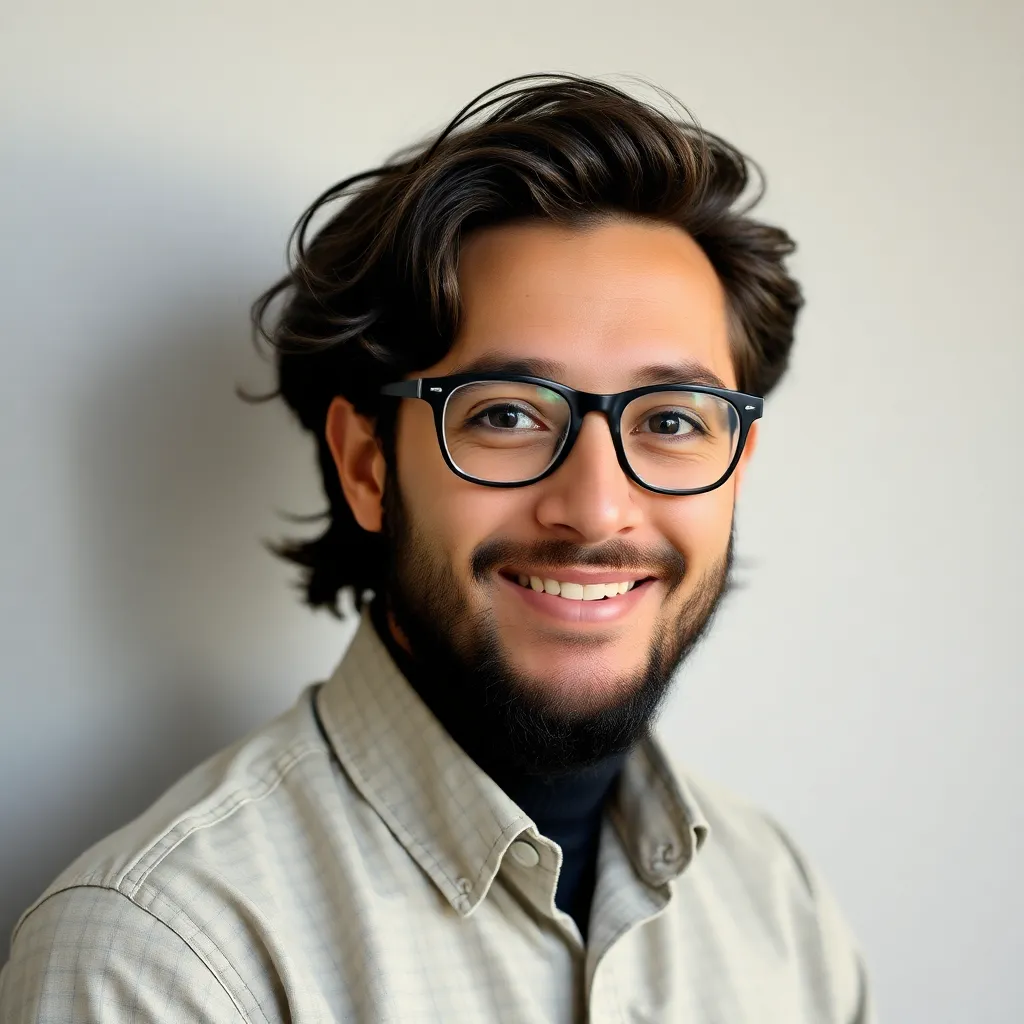
listenit
May 11, 2025 · 6 min read
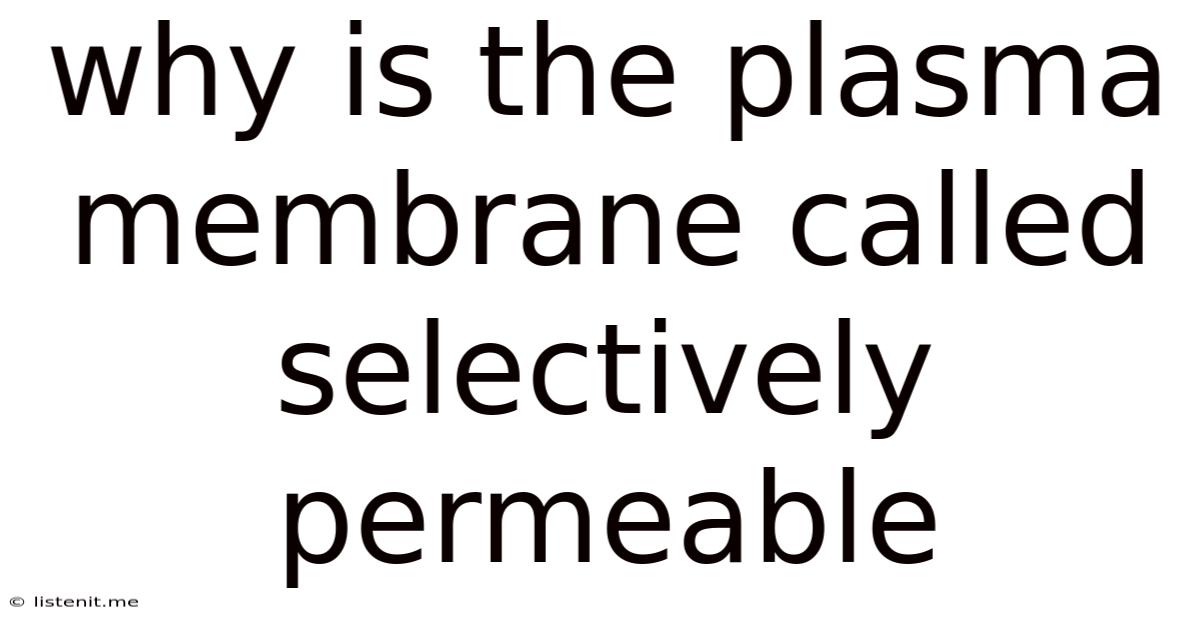
Table of Contents
Why is the Plasma Membrane Called Selectively Permeable?
The plasma membrane, the outer boundary of all cells, isn't just a passive barrier. It's a highly dynamic and sophisticated gatekeeper, meticulously controlling the passage of substances into and out of the cell. This crucial function stems from its selectively permeable nature, a property that allows certain molecules to pass through while excluding others. Understanding this selective permeability is fundamental to comprehending how cells maintain their internal environment, communicate with their surroundings, and ultimately, survive.
The Structure: A Foundation for Selectivity
The selectively permeable nature of the plasma membrane is directly linked to its unique structure, a fluid mosaic model composed primarily of:
1. Phospholipids: The Bilayer Backbone
Phospholipids are amphipathic molecules, meaning they possess both hydrophilic (water-loving) and hydrophobic (water-fearing) regions. This duality is key. The hydrophilic phosphate heads face the watery environments inside and outside the cell, while the hydrophobic fatty acid tails cluster together, forming a central, water-repelling core. This arrangement creates a stable, bilayer structure – a fundamental component underlying the membrane's selective barrier function. The hydrophobic core effectively restricts the passage of many polar molecules and ions, requiring specialized mechanisms for their transport.
2. Proteins: Gateways and Facilitators
Embedded within the phospholipid bilayer are various proteins, performing a multitude of functions. These proteins are crucial for the membrane's selective permeability:
-
Channel Proteins: These proteins form hydrophilic channels or pores through the membrane, allowing specific ions or small polar molecules to pass through passively, following their concentration gradients. Examples include aquaporins, which facilitate the rapid movement of water across the membrane. The specificity of these channels, determined by their size and charge distribution, is essential for selective permeability.
-
Carrier Proteins: These proteins bind to specific molecules on one side of the membrane, undergo a conformational change, and release the molecule on the other side. This facilitated diffusion process, although passive, is still selective because the carrier protein only interacts with specific substrates. Glucose transporters, for instance, selectively facilitate the transport of glucose across the cell membrane.
-
Active Transport Pumps: Unlike channel and carrier proteins involved in passive transport, active transport pumps move molecules against their concentration gradient, requiring energy (usually ATP). This energy-dependent process allows cells to accumulate essential molecules or remove waste products even when their concentrations are higher inside the cell. The sodium-potassium pump, responsible for maintaining the electrochemical gradient across nerve cell membranes, is a prime example.
3. Cholesterol: Maintaining Fluidity and Stability
Cholesterol molecules, interspersed within the phospholipid bilayer, play a vital role in regulating membrane fluidity. At higher temperatures, cholesterol restricts excessive movement of phospholipids, preventing the membrane from becoming too fluid. Conversely, at lower temperatures, cholesterol prevents the phospholipids from packing too tightly, maintaining fluidity and preventing solidification. This fluidity is crucial for membrane function, as it allows proteins to move laterally within the membrane and interact with other molecules.
Mechanisms of Selective Permeability: Passive vs. Active Transport
The movement of substances across the selectively permeable plasma membrane occurs via several mechanisms:
Passive Transport: Following the Gradient
Passive transport processes do not require energy expenditure. Substances move down their concentration gradient, from an area of high concentration to an area of low concentration. Examples include:
-
Simple Diffusion: Small, nonpolar molecules like oxygen and carbon dioxide can readily diffuse across the hydrophobic core of the phospholipid bilayer. Their passage is dictated solely by their concentration gradient.
-
Facilitated Diffusion: As mentioned above, polar molecules and ions utilize channel and carrier proteins to cross the membrane passively. This process is faster than simple diffusion and is highly specific.
-
Osmosis: The passive movement of water across a selectively permeable membrane from a region of high water concentration (low solute concentration) to a region of low water concentration (high solute concentration) is a crucial process for maintaining cellular hydration. The osmotic pressure generated can significantly affect cell volume and function.
Active Transport: Energy-Driven Movement
Active transport mechanisms require energy, usually in the form of ATP, to move substances against their concentration gradient. This is essential for maintaining specific intracellular concentrations of ions and molecules. Key examples include:
-
Primary Active Transport: Directly utilizes ATP to pump molecules against their concentration gradient. The sodium-potassium pump is the classic example.
-
Secondary Active Transport: Uses the energy stored in an electrochemical gradient (created by primary active transport) to move another substance against its concentration gradient. Co-transport and counter-transport are common mechanisms.
The Importance of Selective Permeability: Maintaining Cellular Homeostasis
The selectively permeable nature of the plasma membrane is critical for maintaining cellular homeostasis, the stable internal environment essential for cellular function. This is achieved through:
-
Regulating Ion Concentrations: Maintaining precise intracellular ion concentrations (e.g., potassium, sodium, calcium) is vital for numerous cellular processes, including nerve impulse transmission, muscle contraction, and enzyme activity. The plasma membrane tightly controls the influx and efflux of these ions.
-
Controlling Nutrient Uptake: Cells must selectively absorb essential nutrients and metabolites from their surroundings. The plasma membrane acts as a gatekeeper, allowing the uptake of glucose, amino acids, and other vital molecules while excluding harmful substances.
-
Removing Waste Products: Metabolic waste products must be efficiently expelled from the cell to prevent accumulation and toxicity. The plasma membrane facilitates the removal of carbon dioxide, urea, and other waste products.
-
Cell Signaling: The plasma membrane plays a crucial role in cell signaling, allowing cells to communicate with each other and respond to external stimuli. Receptors embedded in the membrane bind to signaling molecules, triggering intracellular cascades that regulate various cellular functions.
Dysfunction and Disease: When Selectivity Fails
Disruptions in the selective permeability of the plasma membrane can have severe consequences, leading to various diseases and disorders. Examples include:
-
Cystic Fibrosis: A genetic disorder affecting a chloride channel protein, leading to impaired ion transport across the membrane and resulting in thick, sticky mucus in the lungs and other organs.
-
Diabetes Mellitus: Impaired glucose transport across cell membranes, due to defects in insulin signaling or glucose transporters, leads to elevated blood glucose levels.
-
Neurological Disorders: Disruptions in ion channel function can affect nerve impulse transmission, contributing to neurological disorders like epilepsy and myasthenia gravis.
Conclusion: A Dynamic and Essential Barrier
The plasma membrane’s selectively permeable nature is not a static property, but a dynamic process shaped by the complex interplay of its constituent components. Its remarkable ability to control the movement of substances across the cell boundary is fundamental to cell survival and function. A thorough understanding of this selective permeability, its mechanisms, and its implications for health and disease is crucial in various fields, including medicine, biotechnology, and fundamental biological research. Ongoing research continues to unravel the intricacies of membrane function and the precise mechanisms underlying its selective permeability, constantly expanding our knowledge of this essential cellular component.
Latest Posts
Latest Posts
-
What Is The Speed Of Light In Water
May 12, 2025
-
As You Travel Down A Group The Atomic Size
May 12, 2025
-
What Is The Prime Factorization Of 350
May 12, 2025
-
Standard Form Of A Polynomial Example
May 12, 2025
-
Factor The Expression Over The Complex Numbers X2 36
May 12, 2025
Related Post
Thank you for visiting our website which covers about Why Is The Plasma Membrane Called Selectively Permeable . We hope the information provided has been useful to you. Feel free to contact us if you have any questions or need further assistance. See you next time and don't miss to bookmark.