Why Does Ionization Decrease Down A Group
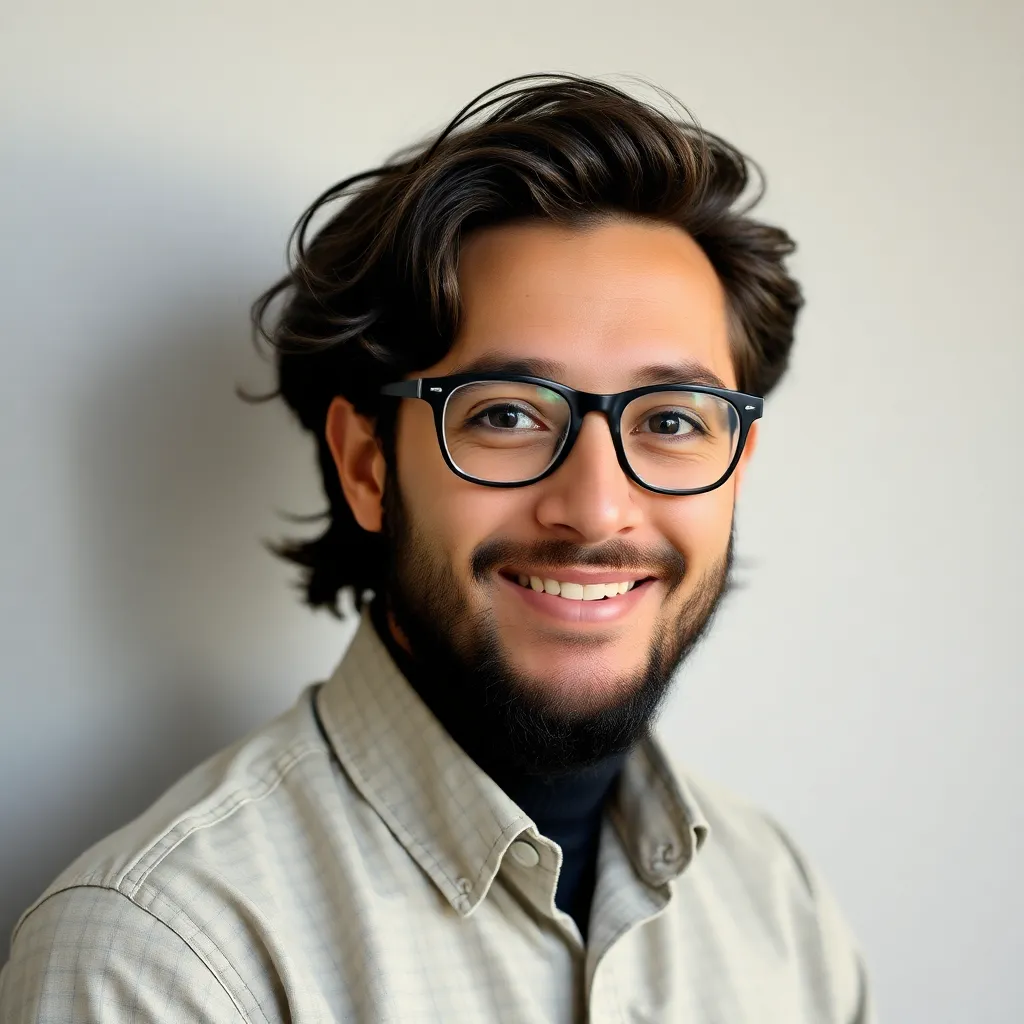
listenit
Apr 02, 2025 · 5 min read
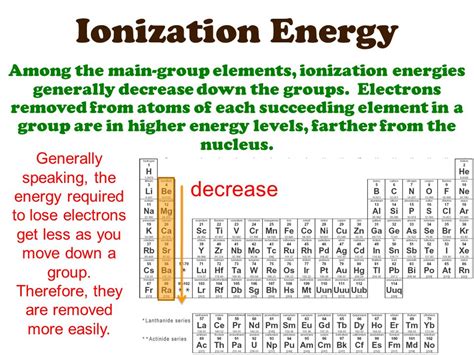
Table of Contents
Why Does Ionization Energy Decrease Down a Group? A Deep Dive into Periodic Trends
Understanding periodic trends is fundamental to grasping the behavior of elements and their interactions. One crucial trend is the decrease in ionization energy down a group in the periodic table. This article delves deep into the reasons behind this phenomenon, exploring the underlying atomic structure and the forces at play. We'll examine the shielding effect, increased atomic radius, and the influence of electron-electron repulsion to provide a comprehensive explanation.
The Fundamentals: What is Ionization Energy?
Before diving into the decrease down a group, let's establish a clear understanding of ionization energy itself. Ionization energy (IE) is the minimum amount of energy required to remove the most loosely bound electron from a neutral gaseous atom. This process transforms a neutral atom into a positively charged ion (cation). The first ionization energy (IE₁) refers to the removal of the first electron, the second ionization energy (IE₂) refers to the removal of the second electron, and so on. Each subsequent ionization energy is progressively larger because removing an electron from a positively charged ion requires overcoming the stronger electrostatic attraction between the remaining electrons and the increasingly positive nucleus.
The Downward Trend: Why Ionization Energy Decreases Down a Group
As we move down a group in the periodic table, the ionization energy generally decreases. This seemingly simple trend arises from a complex interplay of several factors:
1. Increased Atomic Radius: The Distance Factor
The most significant contributor to the decrease in ionization energy down a group is the increase in atomic radius. As we descend a group, a new electron shell is added with each successive element. This leads to a larger distance between the outermost electrons and the positively charged nucleus. The electrostatic force of attraction between the nucleus and the valence electrons weakens significantly with increased distance. Think of it like this: the farther away you are from a magnet, the weaker its pull. Similarly, the weaker attraction allows for easier removal of the outermost electron, hence the lower ionization energy.
2. Shielding Effect: The Electron Shield
The shielding effect, also known as the screening effect, plays a crucial role. Inner electrons, those in shells closer to the nucleus, shield the outer valence electrons from the full positive charge of the nucleus. As we move down a group, the number of inner electrons (core electrons) increases. This increased number of core electrons effectively shields the outermost electrons from the nucleus's pull, further reducing the electrostatic attraction and consequently lowering the ionization energy. The core electrons act like a buffer, reducing the "felt" nuclear charge by the valence electrons.
Illustrative Example: Consider the alkali metals (Group 1). Lithium (Li) has only two inner electrons shielding its single valence electron. Sodium (Na), below lithium, has ten inner electrons shielding its single valence electron. This significantly greater shielding in sodium weakens the attraction between the nucleus and the valence electron, leading to a lower ionization energy compared to lithium.
3. Electron-Electron Repulsion: The Crowded Space
The increased number of electrons in larger atoms also leads to increased electron-electron repulsion. These electrons, particularly the valence electrons, repel each other. This repulsion works against the attractive force of the nucleus, making it easier to remove an electron, thus contributing to the lower ionization energy. The more electrons present, the stronger the repulsion becomes, further facilitating the removal of an outer electron.
4. Effective Nuclear Charge: The Net Attraction
The concept of effective nuclear charge (Z<sub>eff</sub>) helps consolidate the factors above. Z<sub>eff</sub> represents the net positive charge experienced by the valence electrons after accounting for the shielding effect of inner electrons. While the actual nuclear charge increases down a group, the increase in shielding effect causes the effective nuclear charge to increase at a much slower rate. The slower increase in Z<sub>eff</sub>, coupled with the increased distance (atomic radius), results in a net decrease in the attraction between the nucleus and the valence electrons, leading to the observed reduction in ionization energy.
Exceptions and Irregularities: Understanding the Nuances
While the general trend is a decrease in ionization energy down a group, exceptions exist. These irregularities usually stem from subtle variations in electron configurations and inter-electron repulsions. For instance, certain electron configurations can lead to increased stability, requiring more energy to remove an electron. These exceptions highlight the complexity of atomic interactions and the nuanced interplay of the factors discussed above.
Practical Applications and Importance
The understanding of ionization energy and its periodic trends has far-reaching implications across various fields:
-
Chemistry: Ionization energy is crucial for predicting the reactivity of elements. Elements with low ionization energies readily lose electrons, forming cations and exhibiting metallic character. Elements with high ionization energies tend to gain electrons, forming anions and showing non-metallic behavior.
-
Spectroscopy: The ionization energy of an element is directly related to its atomic spectrum. Analyzing the spectral lines provides insights into the energy levels of electrons within the atom and helps determine ionization energies experimentally.
-
Materials Science: The ionization energy is critical in designing and understanding the properties of materials. For instance, understanding the ionization energies of semiconductor materials is vital for developing electronic devices.
-
Astrophysics: The ionization energies of elements help astronomers analyze the composition of stars and nebulae by interpreting their spectral signatures. The degree of ionization of elements in astronomical objects provides clues about their temperature and density.
Conclusion: A Comprehensive Overview
The decrease in ionization energy down a group is a fundamental periodic trend directly linked to the increase in atomic radius, enhanced shielding effect, and increased electron-electron repulsion. These factors combine to reduce the effective nuclear charge experienced by valence electrons, making them easier to remove and leading to the observed trend. While some exceptions exist, the overall pattern is a testament to the elegant and predictable nature of the periodic table and the underlying principles of atomic structure and quantum mechanics. Understanding this trend is not merely an academic exercise; it forms the bedrock of our comprehension of chemical reactivity, spectroscopic analysis, and the properties of materials across various scientific domains. Further exploration into this trend can unlock deeper insights into the intricate world of atomic interactions and their influence on macroscopic properties.
Latest Posts
Latest Posts
-
Does The Pythagorean Theorem Work For All Triangles
Apr 03, 2025
-
Which Would Be Least Likely To Completely Dissolve In Water
Apr 03, 2025
-
In A Nonpolar Covalent Bond Electrons Are
Apr 03, 2025
-
What Is The Oxidation State Of Sulfur In H2so4
Apr 03, 2025
-
How Many Chromosomes Do Cows Have
Apr 03, 2025
Related Post
Thank you for visiting our website which covers about Why Does Ionization Decrease Down A Group . We hope the information provided has been useful to you. Feel free to contact us if you have any questions or need further assistance. See you next time and don't miss to bookmark.