What Is The Molecular Geometry Of N2
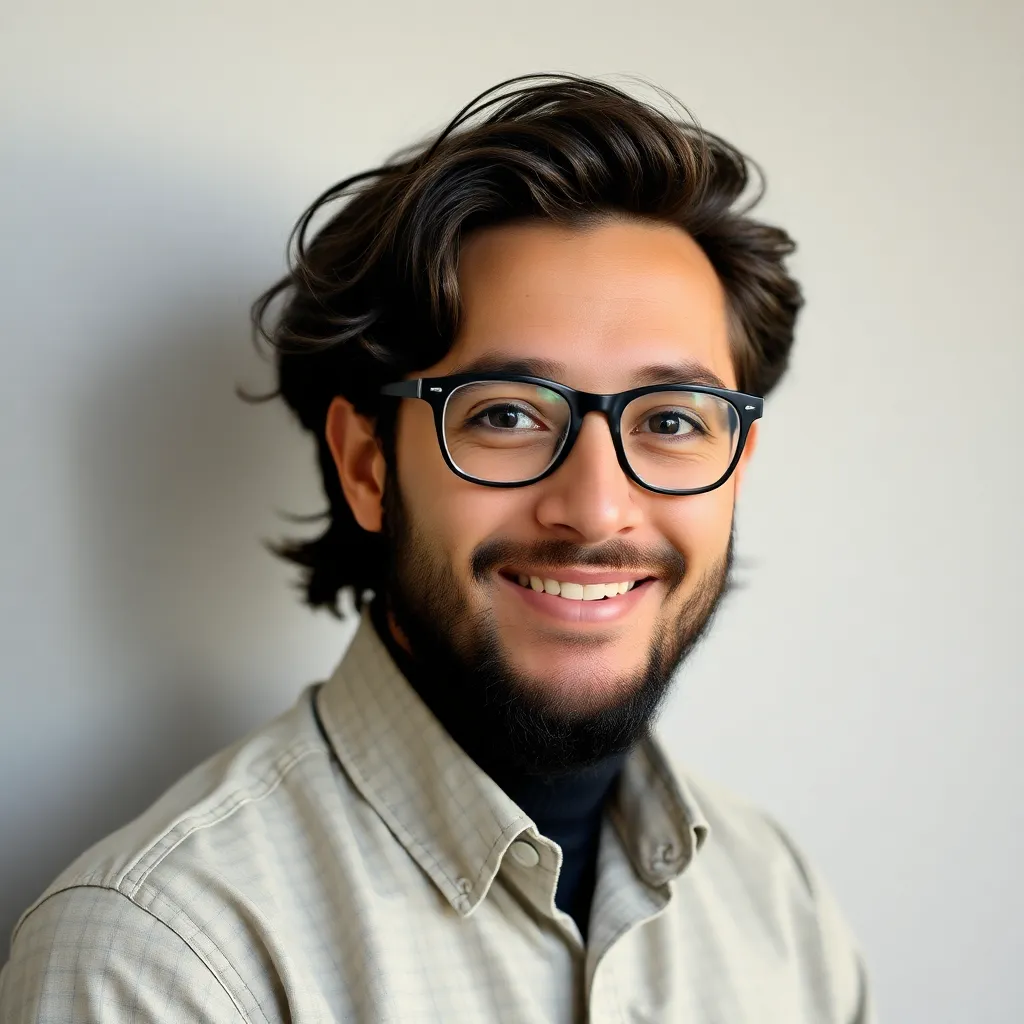
listenit
May 12, 2025 · 5 min read

Table of Contents
What is the Molecular Geometry of N₂? A Deep Dive into Dinitrogen
Nitrogen gas (N₂), a ubiquitous component of Earth's atmosphere, boasts a remarkably simple yet significant molecular structure. Understanding its molecular geometry is crucial for comprehending its chemical behavior and its role in various biological and industrial processes. This article will explore the molecular geometry of N₂, delving into the concepts of bonding, VSEPR theory, and the implications of this linear structure.
Understanding Chemical Bonding in N₂
Before discussing the geometry, let's establish the foundation: the chemical bonds that hold the two nitrogen atoms together. Nitrogen atoms each possess five valence electrons. To achieve a stable octet configuration, they share three pairs of electrons, forming a triple bond. This triple bond consists of one sigma (σ) bond and two pi (π) bonds.
The Significance of the Triple Bond
The triple bond in N₂ is exceptionally strong, contributing to its high bond dissociation energy. This strong bond explains the remarkable stability and inertness of nitrogen gas under standard conditions. Breaking this triple bond requires a significant amount of energy, making it relatively unreactive compared to other elements. This inherent stability is essential for its role in atmospheric processes and its use in various industrial applications.
Sigma and Pi Bonds: A Closer Look
The sigma bond is formed by the direct overlap of atomic orbitals, resulting in electron density concentrated along the internuclear axis. The two pi bonds are formed by the sideways overlap of p-orbitals, leading to electron density above and below the internuclear axis. This combination of sigma and pi bonds creates a strong, stable, and relatively short bond length between the two nitrogen atoms. This unique bonding arrangement directly influences the molecular geometry.
VSEPR Theory and Molecular Geometry Prediction
The Valence Shell Electron Pair Repulsion (VSEPR) theory is a powerful tool for predicting the three-dimensional arrangement of atoms in a molecule. This theory postulates that electron pairs surrounding a central atom repel each other, and they arrange themselves to minimize this repulsion. This minimization leads to specific geometric shapes depending on the number of electron pairs.
Applying VSEPR to N₂
In the case of N₂, each nitrogen atom can be considered a central atom, bonded to the other nitrogen atom. There are no lone pairs of electrons on either nitrogen atom; only the three bonding pairs contributing to the triple bond. According to VSEPR theory, two electron pairs around a central atom will arrange themselves linearly to maximize the distance between them. Therefore, the molecular geometry of N₂ is linear.
The Linear Geometry of N₂: Implications and Properties
The linear geometry of N₂ has several significant implications regarding its physical and chemical properties.
Bond Length and Strength: A Direct Consequence of Geometry
The linear arrangement of atoms optimizes the overlap of atomic orbitals, resulting in a shorter and stronger triple bond. This shorter bond length and higher bond strength directly contribute to the high bond dissociation energy, explaining the inertness of N₂.
Polarity: A Nonpolar Molecule
Due to the symmetrical arrangement of atoms and the identical electronegativity of the nitrogen atoms, the electron density is evenly distributed. This symmetry results in a nonpolar molecule, meaning it has no net dipole moment. This nonpolar nature impacts its interactions with other molecules and its solubility in different solvents.
Physical Properties: Low Boiling and Melting Points
The weak intermolecular forces in N₂, a consequence of its nonpolar nature, lead to relatively low boiling and melting points. This is reflected in its gaseous state at standard temperature and pressure. This low boiling point is crucial for its role in various industrial processes where its gaseous state is vital.
Chemical Inertness and Reactivity: The Role of Geometry and Bonding
The linear geometry and the strong triple bond contribute to the remarkable chemical inertness of N₂ under normal conditions. The high bond dissociation energy prevents spontaneous reactions with many other substances. However, under specific conditions, such as high temperatures or the presence of catalysts, N₂ can participate in reactions, such as the Haber-Bosch process for ammonia synthesis.
Industrial Applications: Harnessing the Properties of N₂
The unique properties of N₂, stemming directly from its molecular geometry and bonding, have led to its widespread use in various industrial applications.
Inert Atmosphere: Protecting Against Oxidation
The inert nature of N₂ makes it ideal for creating an inert atmosphere to protect materials from oxidation. It’s used in packaging to extend the shelf life of food products and in various industrial processes to prevent unwanted reactions.
Cryogenics: Utilizing its Low Boiling Point
The low boiling point of N₂ makes it valuable as a cryogenic refrigerant. Liquid nitrogen is used in various applications, including freezing biological samples, preserving food, and cooling electronic components.
Chemical Synthesis: Ammonia Production
Despite its inherent inertness, N₂ plays a crucial role in the Haber-Bosch process, a high-pressure, high-temperature reaction that produces ammonia (NH₃), a vital component of fertilizers. This process exemplifies how, under specific conditions, the strong triple bond can be broken to facilitate chemical synthesis.
Beyond the Basics: Exploring Advanced Concepts
While the linear geometry of N₂ is a fundamental aspect of its structure, a more comprehensive understanding requires exploring some advanced concepts.
Molecular Orbital Theory (MOT): A Deeper Dive into Bonding
While VSEPR theory successfully predicts the geometry, Molecular Orbital Theory provides a more detailed description of the bonding in N₂. MOT considers the combination of atomic orbitals to form molecular orbitals, leading to a more nuanced understanding of the sigma and pi bonds and their contributions to the overall stability of the molecule.
Vibrational Spectroscopy: Experimental Evidence for Linear Geometry
Infrared (IR) and Raman spectroscopy provide experimental evidence confirming the linear geometry and the vibrational modes of the N₂ molecule. The observed vibrational frequencies align with the theoretical predictions for a linear molecule.
Conclusion: The Importance of Molecular Geometry
The linear molecular geometry of N₂ is not just a theoretical concept; it's a fundamental property that dictates its behavior and applications. Understanding its bonding, the strong triple bond, and the consequences of its linear arrangement helps us comprehend its chemical inertness, its low boiling point, and its wide range of industrial applications. From preserving food to producing fertilizers, the seemingly simple molecule N₂ plays a significant role in various aspects of modern life, highlighting the crucial role of molecular geometry in determining the properties and utility of chemical substances. Further exploration of advanced concepts like MOT and vibrational spectroscopy reinforces our understanding and provides experimental evidence supporting the predicted linear geometry.
Latest Posts
Latest Posts
-
18 Is 60 Percent Of What Number
May 12, 2025
-
How To Solve 5 X 2
May 12, 2025
-
What Would Happen If Photosynthesis Stopped Happening On Earth
May 12, 2025
-
How Many Protons Does Zirconium Have
May 12, 2025
-
Length Of A Polar Curve Formula
May 12, 2025
Related Post
Thank you for visiting our website which covers about What Is The Molecular Geometry Of N2 . We hope the information provided has been useful to you. Feel free to contact us if you have any questions or need further assistance. See you next time and don't miss to bookmark.