What Happens To Water Molecules In Light Reactions
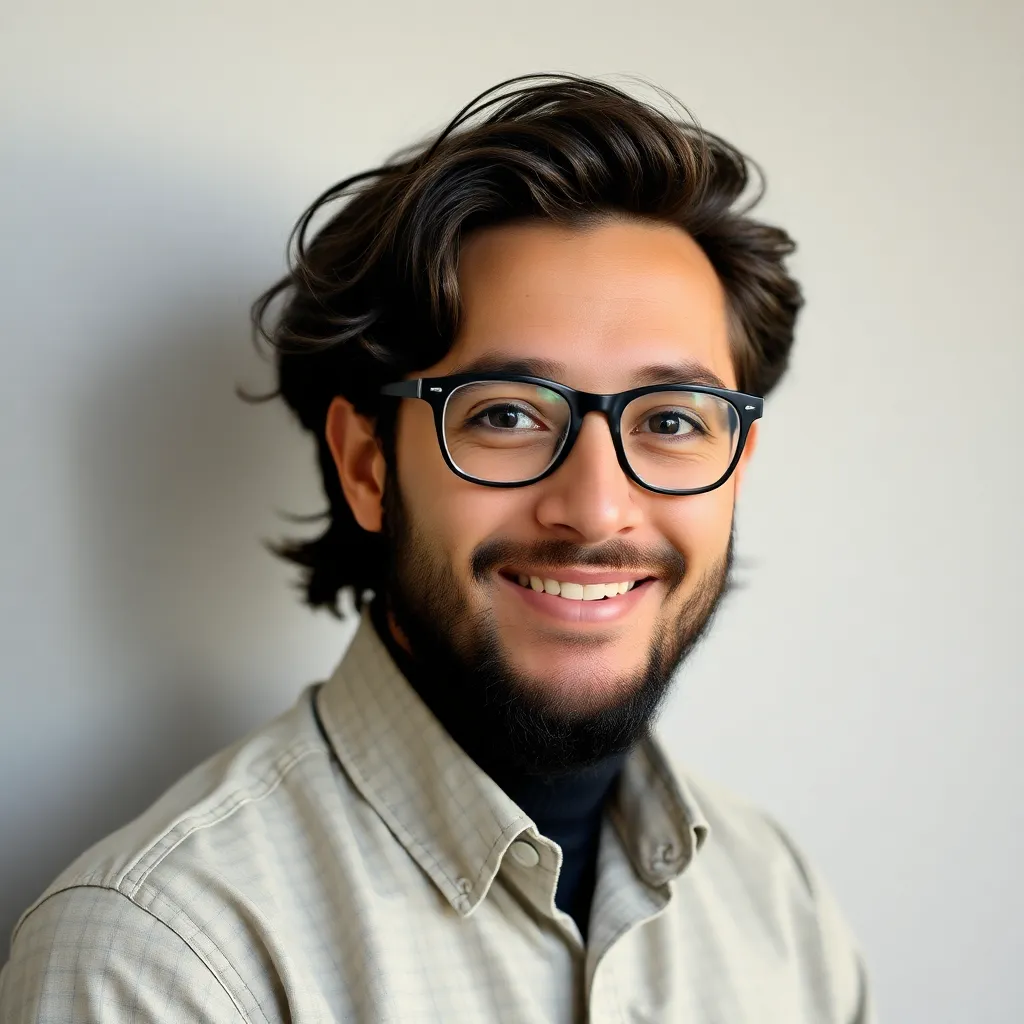
listenit
Apr 03, 2025 · 6 min read
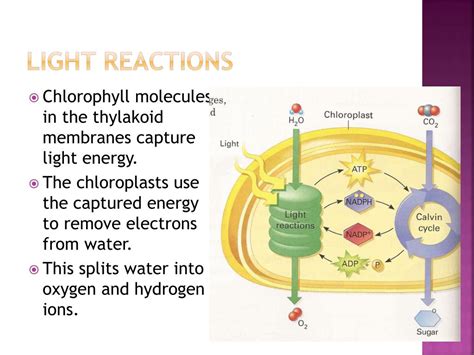
Table of Contents
What Happens to Water Molecules in the Light Reactions of Photosynthesis?
Photosynthesis, the remarkable process by which plants and other organisms convert light energy into chemical energy, is a cornerstone of life on Earth. This intricate process is broadly divided into two main stages: the light-dependent reactions (light reactions) and the light-independent reactions (Calvin cycle). While the Calvin cycle focuses on carbon fixation, the light reactions are where the initial energy capture occurs, and water molecules play a crucial role. Understanding what happens to water molecules during this stage is vital to grasping the overall mechanics of photosynthesis.
The Crucial Role of Water in the Light Reactions
The light reactions, primarily occurring within the thylakoid membranes of chloroplasts, are driven by sunlight. This energy is harnessed to generate ATP (adenosine triphosphate), the cell's energy currency, and NADPH, a reducing agent essential for the subsequent Calvin cycle. Water molecules serve as the primary electron donor in this process, providing the electrons needed to replace those lost by chlorophyll molecules during the excitation process.
Photolysis: The Splitting of Water
The process begins with photolysis, also known as the splitting of water. This is not a spontaneous event; it requires the energy absorbed by chlorophyll and other pigment molecules within the photosystems embedded in the thylakoid membranes. The energy excites electrons in these pigments, initiating a chain of electron transfers that ultimately lead to the reduction of NADP+ to NADPH.
To understand photolysis in detail, we must consider the specific components involved:
-
Photosystem II (PSII): This photosystem plays a pivotal role in water splitting. It contains a manganese cluster that acts as a catalyst for the oxidation of water. The precise mechanism is complex but involves the sequential extraction of electrons from water molecules, eventually releasing oxygen as a byproduct. This is the source of the oxygen we breathe.
-
Manganese Cluster: This complex of manganese ions, along with calcium and chloride ions, acts as the water-oxidizing complex (WOC). It is responsible for extracting four electrons one at a time from two water molecules (2H₂O). This process requires the absorption of four photons of light.
-
Electron Transport Chain: The electrons extracted from water are passed along an electron transport chain, a series of protein complexes embedded in the thylakoid membrane. As electrons move through this chain, energy is released, which is used to pump protons (H+) from the stroma into the thylakoid lumen, creating a proton gradient.
-
Proton Gradient & ATP Synthesis: This proton gradient drives ATP synthesis through chemiosmosis. Protons flow back into the stroma through ATP synthase, an enzyme that uses the energy of the proton flow to synthesize ATP from ADP and inorganic phosphate (Pi).
The Fate of Water's Components: Electrons, Protons, and Oxygen
The splitting of a water molecule (2H₂O) results in the release of several crucial components:
-
Four Electrons (4e⁻): These electrons are essential for replacing those lost by chlorophyll in PSII, maintaining the continuous flow of electrons through the electron transport chain. Without this replenishment, the light reactions would quickly cease.
-
Four Protons (4H⁺): These protons contribute to the proton gradient across the thylakoid membrane. This gradient is crucial for driving ATP synthesis through chemiosmosis, as mentioned earlier. The build-up of protons in the thylakoid lumen creates a significant electrochemical potential.
-
One Oxygen Molecule (O₂): This is a byproduct of photolysis. The release of oxygen into the atmosphere is a significant contribution of photosynthesis to Earth's environment. It's the reason why photosynthetic organisms are often referred to as "oxygen-evolving" organisms.
Beyond the Basics: A Deeper Dive into the Mechanism
The mechanism of water splitting is remarkably complex and highly regulated. Researchers have invested significant effort in unraveling its intricacies, leading to a deeper understanding of the various steps involved.
The Kok Cycle: A Step-by-Step Breakdown
The Kok cycle, named after Bessel Kok, provides a detailed description of the stepwise oxidation of water in PSII. This cycle involves the sequential extraction of four electrons from two water molecules, requiring four photons of light. Each step involves a specific oxidation state of the manganese cluster, culminating in the release of oxygen and the regeneration of the initial state.
The four steps of the Kok cycle can be summarized as follows:
- S₀ state: The resting state of the manganese cluster.
- S₁ state: One electron is extracted from water, and the manganese cluster becomes oxidized.
- S₂ state: A second electron is extracted.
- S₃ state: A third electron is extracted.
- S₄ state: The fourth electron is extracted, leading to the release of oxygen and the regeneration of the S₀ state.
The Role of Accessory Proteins and Cofactors
The process of water splitting isn't solely dependent on the manganese cluster. Several other proteins and cofactors play crucial roles:
-
Tyrosine Z (Yz): This tyrosine residue acts as an intermediary, transferring electrons from the manganese cluster to the chlorophyll molecule P680 in PSII.
-
Calcium (Ca²⁺) and Chloride (Cl⁻) ions: These ions are essential for the proper functioning of the manganese cluster and its catalytic activity.
The Interplay of Light Reactions and the Calvin Cycle
The products of the light reactions, ATP and NADPH, are crucial for the Calvin cycle. The Calvin cycle takes place in the stroma, utilizing the energy stored in ATP and the reducing power of NADPH to convert carbon dioxide (CO₂) into glucose and other organic molecules. This process is crucial for plant growth and the production of biomass. Therefore, the efficient splitting of water in the light reactions directly influences the efficiency of the entire photosynthetic process.
Practical Applications and Future Research
Understanding the intricacies of water splitting in the light reactions has important implications for various fields:
- Biofuel production: Mimicking the natural process of water splitting could provide a sustainable and clean way to produce hydrogen fuel.
- Artificial photosynthesis: Scientists are developing artificial systems that can mimic photosynthesis, potentially providing a renewable source of energy.
- Climate change mitigation: A better understanding of photosynthesis can contribute to efforts to mitigate climate change by enhancing carbon sequestration.
Ongoing research continues to delve deeper into the mechanism of water splitting, employing advanced techniques like X-ray crystallography and spectroscopy. These studies are providing ever-more detailed insights into the structure and function of the water-oxidizing complex and the surrounding protein environment. Unraveling the remaining mysteries of this remarkable process holds the potential to revolutionize various aspects of our lives. The seemingly simple splitting of a water molecule is, in fact, a marvel of biological engineering, essential to the sustenance of life on Earth.
Latest Posts
Latest Posts
-
9 To The Power Of 3 2
Apr 04, 2025
-
Elements In Periodic Table Are Arranged According To Their
Apr 04, 2025
-
What Is The Square Root Of 67
Apr 04, 2025
-
What Is The Gcf Of 24 And 42
Apr 04, 2025
-
What Are The Natural Resources In The Northeast Region
Apr 04, 2025
Related Post
Thank you for visiting our website which covers about What Happens To Water Molecules In Light Reactions . We hope the information provided has been useful to you. Feel free to contact us if you have any questions or need further assistance. See you next time and don't miss to bookmark.