Chair Conformation Of Alpha D Glucopyranose
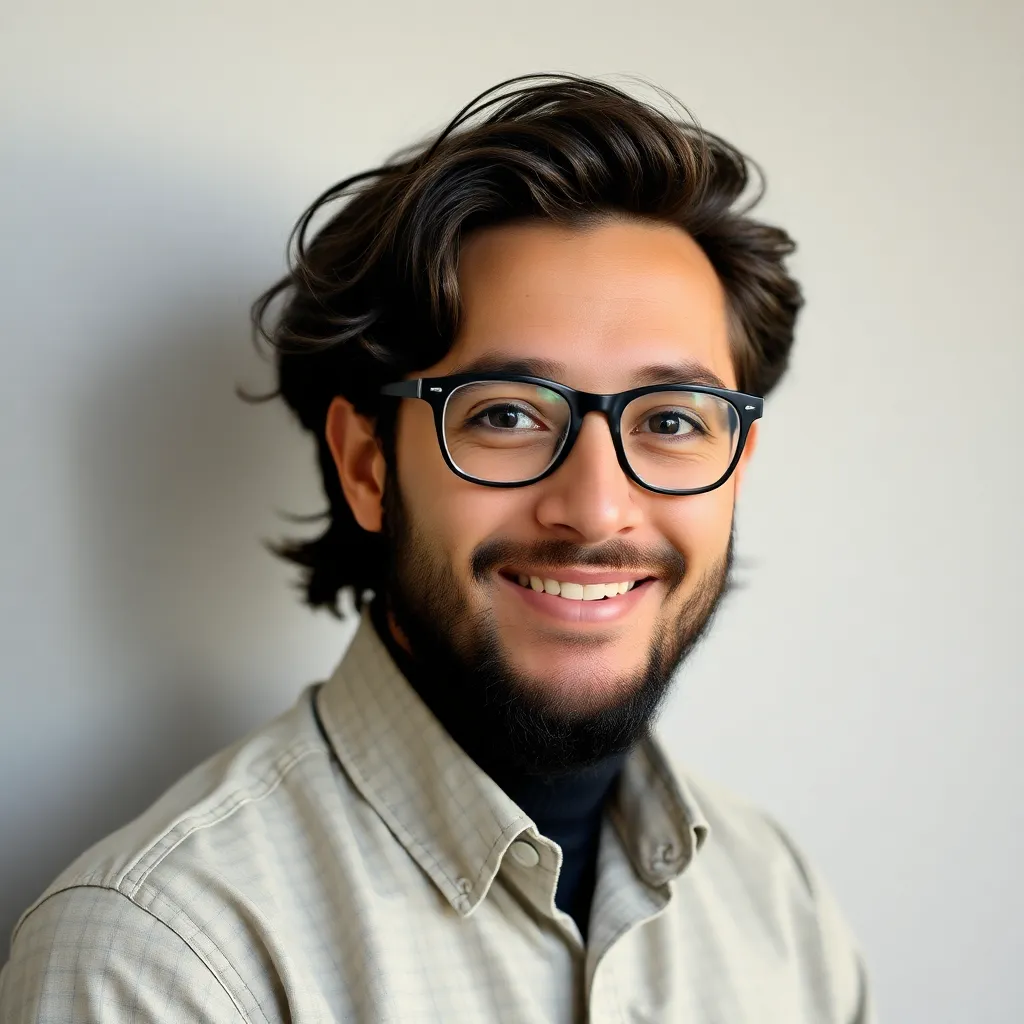
listenit
May 12, 2025 · 5 min read
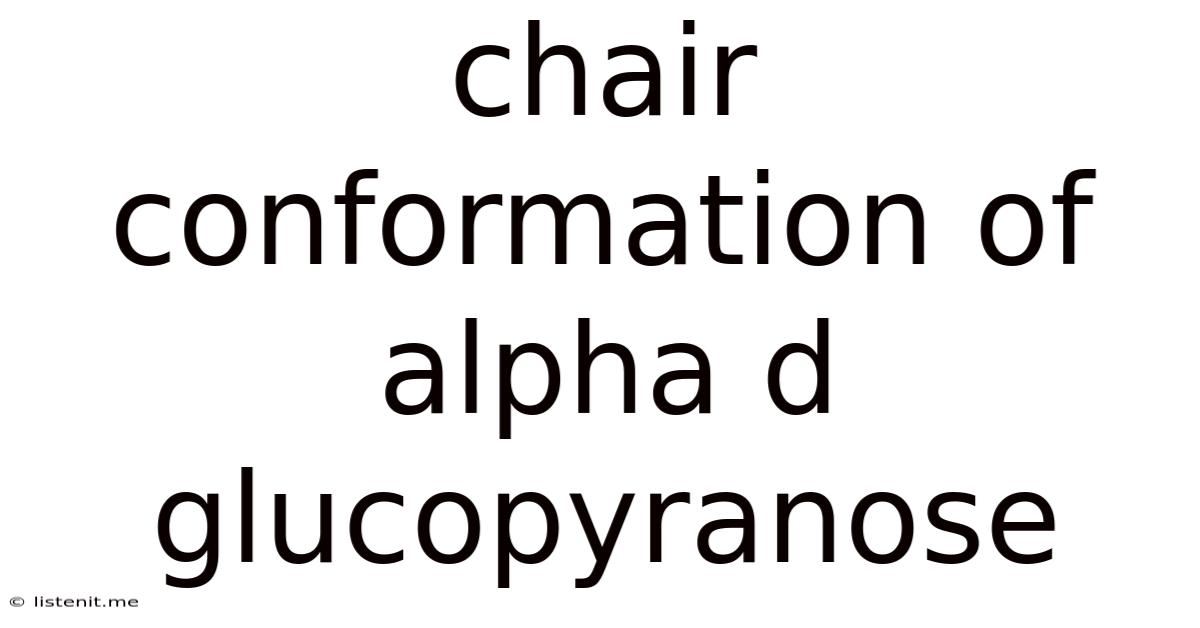
Table of Contents
Chair Conformation of α-D-Glucopyranose: A Deep Dive
The world of carbohydrate chemistry can seem daunting, filled with complex structures and intricate nomenclature. However, understanding the fundamental principles, like the chair conformation of sugars, unlocks a deeper appreciation of their properties and biological roles. This article delves into the intricacies of the chair conformation of α-D-glucopyranose, exploring its stability, substituent effects, and implications for its reactivity and biological function.
Understanding Haworth Projections and Chair Conformations
Before diving into the specifics of α-D-glucopyranose, let's establish a foundational understanding of Haworth projections and chair conformations. Monosaccharides, the simplest carbohydrates, exist predominantly in cyclic forms. These cyclic structures are commonly represented using Haworth projections, which provide a two-dimensional representation of the three-dimensional ring structure. However, Haworth projections are somewhat limited in accurately portraying the true three-dimensional shape.
The reality is that pyranose rings (six-membered rings) adopt a more stable chair conformation. This conformation minimizes steric hindrance between substituents on the ring, leading to greater stability. In a chair conformation, the ring carbons are not planar; instead, they adopt a three-dimensional structure resembling a chair. Each carbon atom in the ring has two substituents: one axial and one equatorial.
Axial substituents are oriented perpendicular to the plane of the ring, pointing either up or down. Equatorial substituents lie roughly in the plane of the ring. The difference in spatial orientation of axial and equatorial substituents has profound implications for the molecule's stability and reactivity.
The Chair Conformation of α-D-Glucopyranose: A Detailed Look
α-D-glucopyranose, a crucial monosaccharide and building block for many complex carbohydrates like starch and cellulose, predominantly exists in a chair conformation. Let's examine its structure in detail:
Identifying Axial and Equatorial Substituents
In the most stable chair conformation of α-D-glucopyranose, the following substituents are observed:
- Anomeric carbon (C1): The α-anomer denotes the hydroxyl group on the anomeric carbon (C1) being positioned axially and downwards.
- C2 hydroxyl group: Positioned equatorially.
- C3 hydroxyl group: Positioned equatorially.
- C4 hydroxyl group: Positioned equatorially.
- C5 hydroxyl group: Positioned axially and upwards.
- C6 – CH₂OH group: Positioned equatorially.
Stability of the Chair Conformation
The preference for the chair conformation over other possible conformations, such as the boat or twist-boat conformations, stems primarily from the minimization of steric clashes between substituents. In boat and twist-boat conformations, bulky substituents experience significant steric hindrance, leading to instability. The chair conformation, with its staggered arrangement of substituents, significantly reduces these interactions.
The specific arrangement of equatorial and axial groups in α-D-glucopyranose further enhances its stability. The bulky hydroxyl groups are predominantly located in equatorial positions, minimizing 1,3-diaxial interactions which are significant sources of steric strain.
Anomeric Effect
An important aspect influencing the stability of α-D-glucopyranose's chair conformation is the anomeric effect. This effect refers to the preference for an electronegative substituent (like an oxygen atom in a hydroxyl group) at the anomeric carbon (C1) to adopt an axial orientation rather than an equatorial orientation. This counter-intuitive preference stems from the interaction of the lone pair electrons on the oxygen atom with the antibonding sigma orbital (σ*) of the C1-O5 bond. This interaction stabilizes the molecule, and while this effect is significant, in α-D-glucopyranose, the predominance of equatorial substituents still favors the chair conformation depicted above.
Consequences of Chair Conformation: Reactivity and Biological Implications
The specific chair conformation of α-D-glucopyranose directly impacts its reactivity and biological functions.
Reactivity
The accessibility of hydroxyl groups plays a crucial role in the reactivity of α-D-glucopyranose. Hydroxyl groups in equatorial positions are more readily accessible to reactants than those in axial positions. This accessibility difference influences the rate of glycosidic bond formation and other reactions involving hydroxyl groups. For instance, the equatorial orientation of many of the hydroxyl groups facilitates enzyme binding and catalysis.
Biological Implications: Starch and Cellulose
The different chair conformations of α- and β-D-glucose are key to the differences in their polymeric forms, starch and cellulose, respectively. α-D-glucopyranose monomers link together through α-1,4-glycosidic bonds to form amylose and amylopectin (components of starch), resulting in a helical structure. In contrast, β-D-glucopyranose monomers, which adopt a slightly different chair conformation with the anomeric hydroxyl group in the equatorial position, link through β-1,4-glycosidic bonds to form cellulose, leading to a linear, fiber-like structure. These structural differences dramatically affect the digestibility and physical properties of starch and cellulose.
Conformational Changes and Dynamics
While the chair conformation is predominantly favored, it is important to remember that it's not static. The molecule undergoes conformational changes, though these occur on a relatively short timescale, influencing reactivity and interactions with enzymes. These subtle fluctuations and interconversion between chair conformations are vital aspects in various enzymatic processes involving glucopyranose.
Factors Affecting Conformational Equilibrium
Several factors can influence the equilibrium between different chair conformations of α-D-glucopyranose, including temperature, solvent, and the presence of other molecules. Changes in these factors can alter the relative populations of different conformations.
Advanced Concepts and Future Research
Further exploration of the chair conformation of α-D-glucopyranose can involve sophisticated computational techniques, such as molecular dynamics simulations and quantum mechanical calculations. These methods can provide detailed insights into the energetic landscape, dynamics, and interactions governing its conformational behavior. Research continues to refine our understanding of the subtle influences on these conformations and their impact on biological systems. This includes the role of solvent effects, interactions with other biomolecules and exploration of novel conformations and their stability under different conditions.
Conclusion
The chair conformation of α-D-glucopyranose is a pivotal structural feature dictating its chemical reactivity and biological roles. Its specific arrangement of axial and equatorial substituents, influenced by the anomeric effect and steric interactions, defines its stability and influences its participation in numerous biochemical processes. Understanding this conformation provides a crucial foundation for appreciating the complexities of carbohydrate chemistry and the biological functions of this vital monosaccharide. Further research will continue to unveil the subtle intricacies of its conformational dynamics and impact on biological systems.
Latest Posts
Latest Posts
-
What Is 5 Out Of 12 As A Percentage
May 12, 2025
-
Why Are Covalent Bonds Stronger Than Ionic
May 12, 2025
-
What Is 40 Percent Off Of 60
May 12, 2025
-
What Is The Basic Structural And Functional Unit Of Life
May 12, 2025
-
How Do You Convert 2 5 Into A Decimal
May 12, 2025
Related Post
Thank you for visiting our website which covers about Chair Conformation Of Alpha D Glucopyranose . We hope the information provided has been useful to you. Feel free to contact us if you have any questions or need further assistance. See you next time and don't miss to bookmark.