Write The Balanced Chemical Equation For Cellular Respiration
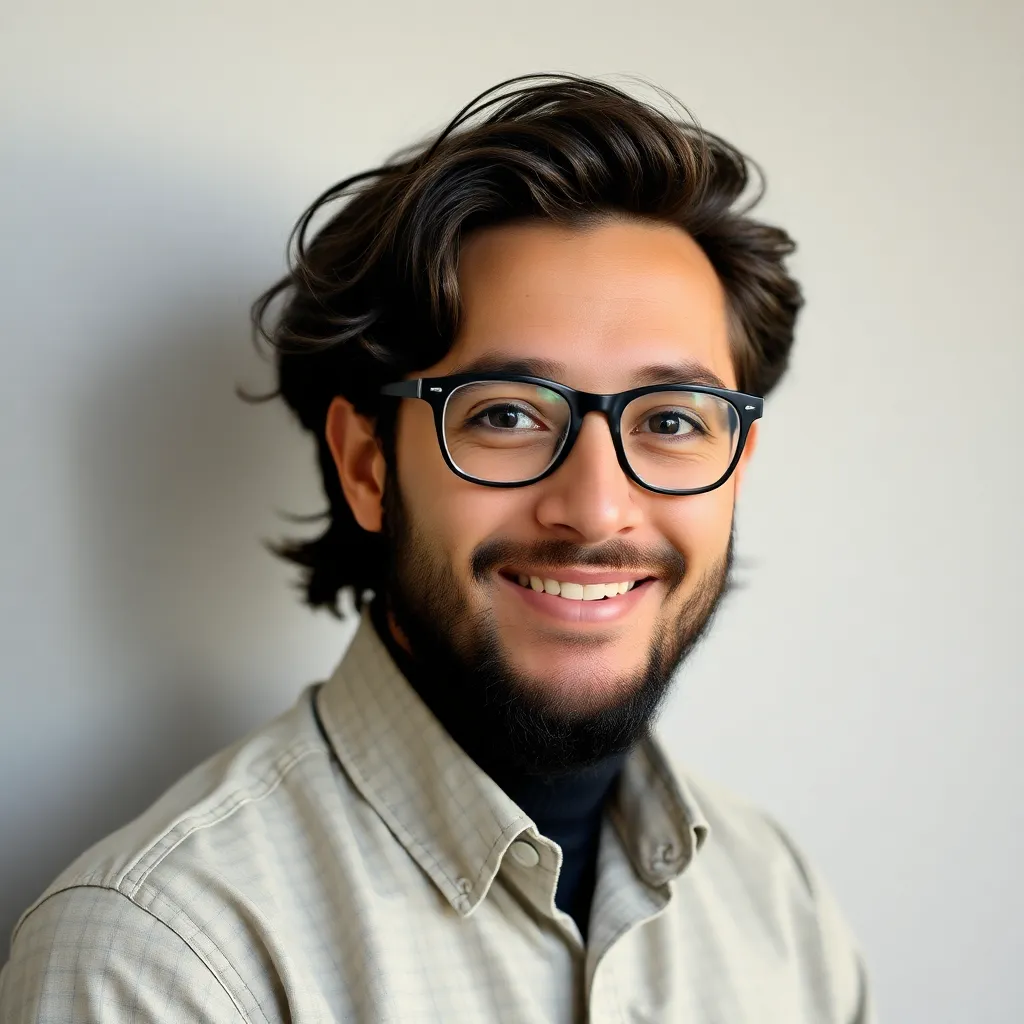
listenit
Apr 11, 2025 · 5 min read

Table of Contents
The Balanced Chemical Equation for Cellular Respiration: A Deep Dive
Cellular respiration is the fundamental process by which living organisms convert the chemical energy stored in glucose into a readily usable form of energy called ATP (adenosine triphosphate). This intricate process is essential for life, powering everything from muscle contractions to protein synthesis. While often simplified in introductory biology texts, a complete understanding requires examining the balanced chemical equation and the nuanced steps involved. This article will delve into the complexities of cellular respiration, exploring the balanced equation, its variations depending on the organism and conditions, and the significance of each component.
Understanding the Simplified Equation
The most common, simplified representation of cellular respiration is:
C₆H₁₂O₆ + 6O₂ → 6CO₂ + 6H₂O + Energy (ATP)
This equation shows glucose (C₆H₁₂O₆) reacting with oxygen (O₂) to produce carbon dioxide (CO₂), water (H₂O), and energy in the form of ATP. However, this simplification obscures the multi-step nature of the process and doesn't accurately reflect the entire energy yield. The true equation is far more complex and involves numerous intermediate reactions.
The Detailed Breakdown: Glycolysis, Krebs Cycle, and Oxidative Phosphorylation
Cellular respiration is not a single reaction but a series of interconnected metabolic pathways:
1. Glycolysis: The First Steps
Glycolysis, meaning "sugar splitting," takes place in the cytoplasm and doesn't require oxygen (anaerobic). It begins with a single molecule of glucose and, through a series of ten enzyme-catalyzed reactions, breaks it down into two molecules of pyruvate (pyruvic acid). This process generates a small amount of ATP (net gain of 2 ATP) and NADH, a crucial electron carrier.
The balanced equation for glycolysis is:
C₆H₁₂O₆ + 2NAD⁺ + 2ADP + 2Pᵢ → 2C₃H₄O₃ + 2NADH + 2ATP + 2H₂O
Where:
- C₆H₁₂O₆: Glucose
- 2NAD⁺: Nicotinamide adenine dinucleotide (oxidized form)
- 2ADP: Adenosine diphosphate
- 2Pᵢ: Inorganic phosphate
- 2C₃H₄O₃: Pyruvate
- 2NADH: Nicotinamide adenine dinucleotide (reduced form)
- 2ATP: Adenosine triphosphate
- 2H₂O: Water
2. The Krebs Cycle (Citric Acid Cycle): Further Oxidation
If oxygen is present (aerobic conditions), pyruvate enters the mitochondria, where it undergoes a series of reactions in the Krebs cycle. Before entering the cycle, pyruvate is converted into acetyl-CoA, releasing CO₂ and producing NADH. The Krebs cycle itself involves a cyclical series of reactions that further oxidize the acetyl-CoA, releasing more CO₂, generating ATP (small amount), NADH, and FADH₂ (another electron carrier).
The overall balanced equation for the Krebs cycle (per glucose molecule, considering two pyruvate molecules) is approximately:
2C₃H₄O₃ + 6NAD⁺ + 2FAD + 2ADP + 2Pᵢ + 6H₂O → 6CO₂ + 6NADH + 2FADH₂ + 2ATP + 8H⁺
Where:
- 2C₃H₄O₃: Pyruvate (from glycolysis)
- 6NAD⁺: Nicotinamide adenine dinucleotide (oxidized form)
- 2FAD: Flavin adenine dinucleotide (oxidized form)
- 2ADP: Adenosine diphosphate
- 2Pᵢ: Inorganic phosphate
- 6CO₂: Carbon dioxide
- 6NADH: Nicotinamide adenine dinucleotide (reduced form)
- 2FADH₂: Flavin adenine dinucleotide (reduced form)
- 2ATP: Adenosine triphosphate
- 8H⁺: Hydrogen ions
3. Oxidative Phosphorylation: ATP Synthesis
Oxidative phosphorylation, occurring in the inner mitochondrial membrane, is where the majority of ATP is produced. The electron carriers NADH and FADH₂, generated during glycolysis and the Krebs cycle, donate their electrons to the electron transport chain (ETC). As electrons move down the ETC, energy is released and used to pump protons (H⁺) across the inner mitochondrial membrane, creating a proton gradient. This gradient drives ATP synthesis through chemiosmosis, using ATP synthase. Oxygen acts as the final electron acceptor, combining with protons and electrons to form water.
The exact balanced equation for oxidative phosphorylation is difficult to represent concisely due to the complexity of the ETC. However, the overall process can be summarized as:
NADH + FADH₂ + O₂ + ADP + Pᵢ → NAD⁺ + FAD + H₂O + ATP
This equation simplifies the extensive electron transfers and proton pumping. The actual ATP yield depends on the efficiency of the ETC and varies slightly depending on the organism and conditions.
The Complete (Approximate) Balanced Equation
Combining the approximate balanced equations for glycolysis, the Krebs cycle, and oxidative phosphorylation, we get a more comprehensive (though still simplified) equation for cellular respiration:
C₆H₁₂O₆ + 6O₂ + 36ADP + 36Pᵢ → 6CO₂ + 6H₂O + 36ATP
Important Note: The actual ATP yield is highly variable. While the theoretical maximum ATP yield from one glucose molecule is often cited as 38 ATP (in eukaryotic cells), the actual yield is usually closer to 30-32 ATP due to factors like the energy cost of transporting NADH into the mitochondria and proton leak across the membrane.
Variations in Cellular Respiration
The balanced equation for cellular respiration can vary depending on the type of organism and the conditions.
-
Anaerobic Respiration: In the absence of oxygen, some organisms utilize anaerobic respiration, which involves alternative electron acceptors instead of oxygen. This process produces less ATP than aerobic respiration, and the end products vary depending on the specific pathway used (e.g., lactic acid fermentation, alcoholic fermentation).
-
Variations in Electron Transport Chain Efficiency: The efficiency of the electron transport chain can vary between organisms and even within different cell types of the same organism.
-
Substrate-Level Phosphorylation: Although oxidative phosphorylation is the primary means of ATP production in cellular respiration, a small amount of ATP is also produced through substrate-level phosphorylation during glycolysis and the Krebs cycle.
-
Alternative Substrates: While glucose is the most common substrate for cellular respiration, other molecules like fatty acids and amino acids can also be broken down and used to generate ATP through various pathways that integrate with the core stages of cellular respiration.
Conclusion
The balanced chemical equation for cellular respiration is a fundamental concept in biology, representing the remarkable energy conversion process crucial for life. While simplified equations provide a general overview, a deeper understanding requires appreciating the multi-step nature of the process, the involvement of various intermediate molecules, and the potential variations based on the organism and environmental conditions. The balanced equations presented here offer a more detailed perspective on this complex and vital metabolic pathway. Remember that these equations represent approximations, and the actual yields and specific reactions can be far more nuanced. This complex interplay of reactions ensures a constant supply of energy to power the myriad functions of living cells.
Latest Posts
Latest Posts
-
What Is The Gcf Of 30 And 60
Apr 18, 2025
-
A Quadrilateral With 2 Right Angles
Apr 18, 2025
-
Distinguish Between Linear Momentum And Angular Momentum
Apr 18, 2025
-
How Do Reproductive Barriers Relate To The Biological Species Concept
Apr 18, 2025
-
16 To The Power Of 3 4 As A Fraction
Apr 18, 2025
Related Post
Thank you for visiting our website which covers about Write The Balanced Chemical Equation For Cellular Respiration . We hope the information provided has been useful to you. Feel free to contact us if you have any questions or need further assistance. See you next time and don't miss to bookmark.