Why Plasma Membrane Is Called Fluid Mosaic
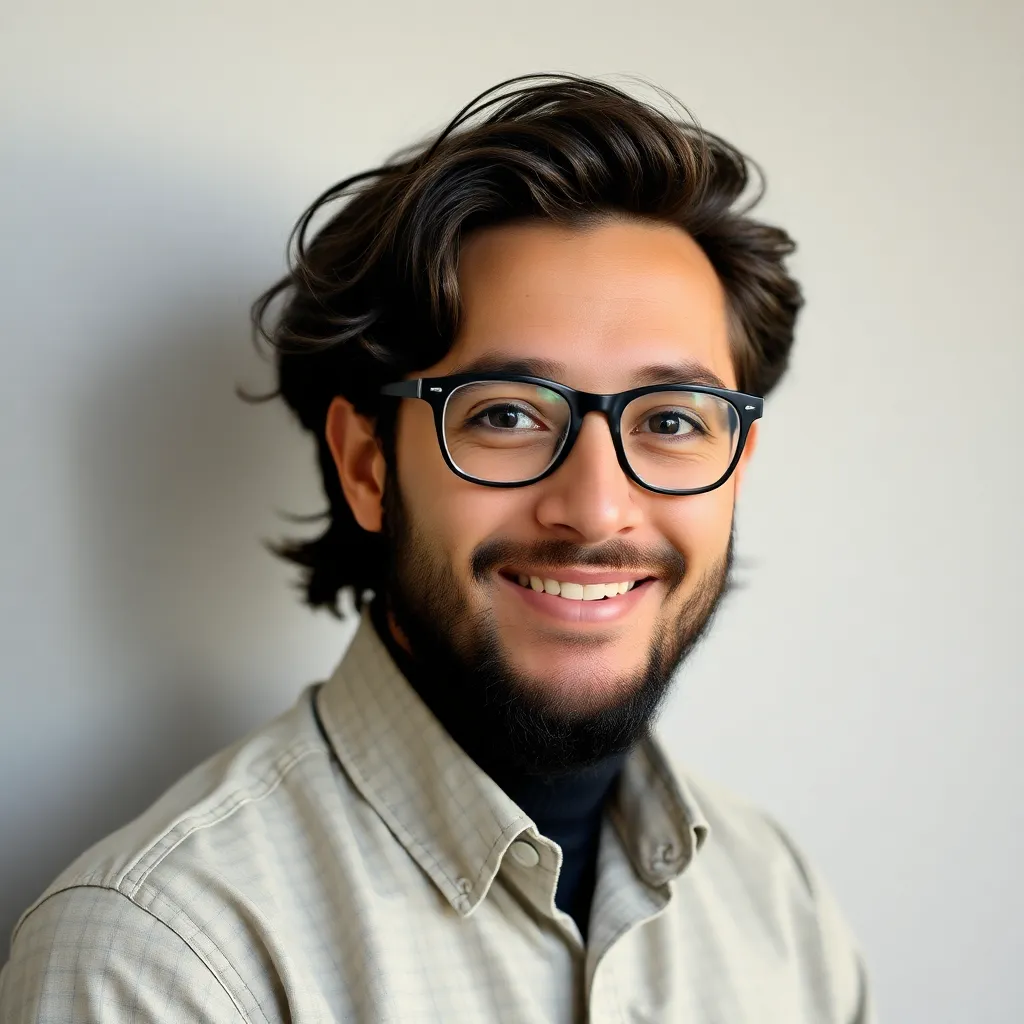
listenit
Apr 08, 2025 · 6 min read

Table of Contents
Why the Plasma Membrane is Called a Fluid Mosaic: A Deep Dive into Cellular Structure
The plasma membrane, that ubiquitous boundary separating the internal environment of a cell from its surroundings, isn't just a static barrier. It's a dynamic, ever-changing structure, constantly adapting to the cell's needs. This dynamic nature is perfectly captured in the term "fluid mosaic model," a description that beautifully encapsulates the membrane's composition and behavior. This article will explore the reasons behind this designation, delving deep into the components and properties that make the plasma membrane such a fascinating and crucial element of life.
The "Fluid" Aspect: A Sea of Lipids
The fluidity of the plasma membrane is primarily dictated by its lipid bilayer. This bilayer isn't a rigid, fixed structure but rather a dynamic sea of phospholipids, cholesterol, and glycolipids, constantly shifting and moving. Let's examine these components individually:
Phospholipids: The Foundation of Fluidity
Phospholipids are amphipathic molecules, meaning they possess both hydrophilic (water-loving) and hydrophobic (water-fearing) regions. This crucial characteristic is what drives the formation of the bilayer. The hydrophilic phosphate heads face outwards, interacting with the aqueous environments inside and outside the cell, while the hydrophobic fatty acid tails cluster together in the interior of the bilayer, avoiding contact with water.
The degree of saturation of the fatty acid tails significantly influences membrane fluidity. Unsaturated fatty acids, with their double bonds creating kinks in their structure, prevent tight packing, leading to increased fluidity. Conversely, saturated fatty acids pack more tightly, reducing fluidity. This is why organisms living in cold environments often have a higher proportion of unsaturated fatty acids in their membranes – maintaining fluidity at low temperatures.
Cholesterol: The Fluidity Buffer
Cholesterol, another crucial lipid component, acts as a fluidity buffer. At high temperatures, it restricts the movement of phospholipids, reducing fluidity and preventing the membrane from becoming too permeable. Conversely, at low temperatures, it prevents the phospholipids from packing too tightly, maintaining a degree of fluidity and preventing the membrane from solidifying. Cholesterol's influence on membrane fluidity is a testament to the membrane's delicate balance and its adaptability to diverse temperature ranges.
Glycolipids: More Than Just Structure
Glycolipids are lipids with attached carbohydrate groups. While their contribution to overall fluidity is less significant compared to phospholipids and cholesterol, they play crucial roles in cell recognition and signaling. These sugar moieties extend outwards from the cell surface, acting as markers for cell-to-cell communication and interaction. They are particularly important in the immune system, allowing immune cells to distinguish between "self" and "non-self" cells.
The "Mosaic" Aspect: A Diverse Collection of Proteins
The fluidity of the lipid bilayer provides the canvas for the mosaic of proteins embedded within it. These proteins are not static fixtures; they move laterally within the membrane, though at a slower rate than the lipids. Their diverse functions are central to the membrane's role as a dynamic interface with the external world. We can categorize membrane proteins broadly into two types:
Integral Membrane Proteins: Embedded within the Bilayer
Integral membrane proteins are firmly embedded within the lipid bilayer, often spanning the entire membrane (transmembrane proteins). They possess hydrophobic regions that interact with the fatty acid tails and hydrophilic regions that interact with the aqueous environments. These proteins perform a variety of functions:
-
Transport Proteins: These proteins facilitate the movement of ions and molecules across the membrane, either passively (channels, carriers) or actively (pumps). Their specific structures allow them to selectively transport particular substances, regulating the cell's internal environment.
-
Receptor Proteins: These proteins bind to specific signaling molecules (ligands), triggering intracellular responses. They play critical roles in cell communication and signaling pathways.
-
Enzymes: Some integral membrane proteins act as enzymes, catalyzing reactions within or near the membrane. Their location within the membrane can facilitate interactions with substrates and products.
-
Structural Proteins: These proteins contribute to the overall structural integrity of the membrane, connecting the cytoskeleton to the extracellular matrix.
Peripheral Membrane Proteins: Associated with the Bilayer Surface
Peripheral membrane proteins are loosely associated with the membrane surface, either binding to integral proteins or interacting with the polar heads of phospholipids. These proteins are more easily detached from the membrane than integral proteins and often play roles in cell signaling and structural support. They are involved in processes such as:
-
Cell Signaling: Many peripheral proteins participate in signal transduction pathways, relaying information from the extracellular environment to the cell interior.
-
Cytoskeletal Attachment: Some peripheral proteins link the membrane to the underlying cytoskeleton, providing structural support and maintaining cell shape.
-
Enzyme Activity: Peripheral proteins can also possess enzymatic activity, playing a role in various metabolic processes.
The Dynamic Nature of the Mosaic: Constant Change and Adaptation
The fluid mosaic model emphasizes the dynamic nature of the membrane. The components are not static; they are constantly moving, interacting, and rearranging. This dynamic nature is crucial for several cellular processes:
-
Membrane Trafficking: The membrane is constantly being synthesized and recycled. Vesicles bud off from the Golgi apparatus and fuse with the plasma membrane, delivering new proteins and lipids. Conversely, sections of the plasma membrane can be internalized via endocytosis, forming vesicles that carry molecules into the cell.
-
Cell Signaling: The fluidity of the membrane allows for the rapid movement and clustering of receptor proteins, facilitating efficient signal transduction.
-
Cell Growth and Division: The membrane's dynamic nature is essential for cell growth and division. The membrane expands during cell growth and is carefully partitioned between daughter cells during division.
-
Cell Migration: The fluidity of the membrane is also crucial for cell migration, enabling the remodeling of the cell shape and the extension of cellular protrusions.
The Importance of the Fluid Mosaic Model
The fluid mosaic model is more than just a descriptive term; it's a fundamental concept that underpins our understanding of cell biology. It explains a range of cellular processes, from membrane transport and signaling to cell growth and division. The dynamic nature of the membrane, with its constant movement and interaction of components, is critical for the cell's survival and function. Without this fluidity and mosaic arrangement, the cell would be unable to adapt to its environment, communicate with other cells, or maintain its integrity.
Furthermore, understanding the fluid mosaic model has significant implications in medicine and biotechnology. Many diseases are linked to defects in membrane structure or function. For instance, mutations in membrane proteins can lead to various genetic disorders, affecting transport processes, signaling pathways, and cell adhesion. Researchers are actively exploring the potential of manipulating membrane properties for therapeutic purposes, including drug delivery and gene therapy.
In conclusion, the term "fluid mosaic model" is a fitting and accurate description of the plasma membrane. The fluidity, arising from the dynamic lipid bilayer, provides the framework for a diverse array of proteins that form a functional mosaic. This dynamic interaction between lipids and proteins is essential for the membrane’s role as a selective barrier and a dynamic interface with the external world. The fluid mosaic model continues to be a cornerstone of cell biology, providing a framework for understanding cellular function and informing advances in medicine and biotechnology.
Latest Posts
Latest Posts
-
Why Does Metal Feel Colder Than Wood
Apr 17, 2025
-
7 12 Divided By 2 9
Apr 17, 2025
-
Lim Of X As X Approaches 0
Apr 17, 2025
-
Is Water Evaporating A Chemical Reaction
Apr 17, 2025
-
18 As A Fraction In Simplest Form
Apr 17, 2025
Related Post
Thank you for visiting our website which covers about Why Plasma Membrane Is Called Fluid Mosaic . We hope the information provided has been useful to you. Feel free to contact us if you have any questions or need further assistance. See you next time and don't miss to bookmark.