Why Is A Cell Membrane Called A Fluid Mosaic
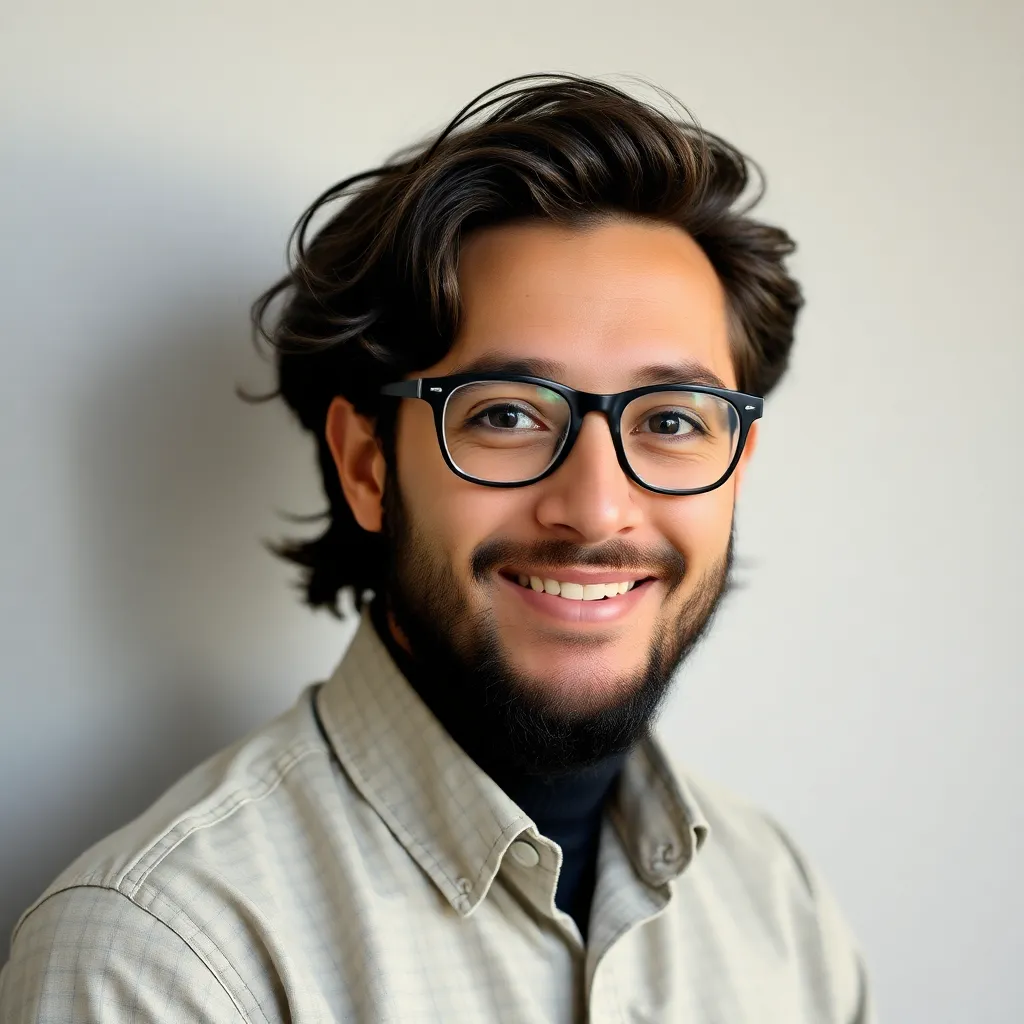
listenit
Apr 18, 2025 · 6 min read

Table of Contents
Why is a Cell Membrane Called a Fluid Mosaic?
The cell membrane, a ubiquitous structure in all living organisms, is far more than just a simple barrier separating the inside of a cell from its surroundings. It's a dynamic, complex entity crucial for cell survival and function. Its descriptive name, the "fluid mosaic model," perfectly encapsulates its multifaceted nature, highlighting both its fluidity and its mosaic-like composition. This article delves deep into the reasons behind this nomenclature, exploring the structural components and dynamic properties that make the cell membrane such a fascinating and vital part of cellular biology.
The "Fluid" Aspect: A Sea of Lipids
The fluidity of the cell membrane is primarily attributed to its lipid bilayer. This bilayer isn't a rigid, static structure; instead, it's a dynamic, constantly shifting sea of phospholipids. These phospholipids are amphipathic molecules, meaning they possess both hydrophilic (water-loving) and hydrophobic (water-fearing) regions.
Phospholipid Bilayer Dynamics
-
Lateral Movement: Phospholipids can easily move laterally within their own leaflet (monolayer) of the bilayer. They're constantly swapping places with their neighbors, a process crucial for membrane fluidity and function. This lateral diffusion is rapid, allowing for the quick rearrangement of membrane components.
-
Flip-Flop Movement: While less frequent than lateral movement, phospholipids can also "flip-flop" between the inner and outer leaflets of the bilayer. This transbilayer movement is facilitated by specific enzymes called flippases, floppases, and scramblases. This process plays a role in maintaining the asymmetrical distribution of lipids between the two leaflets.
-
Factors Affecting Fluidity: Several factors influence the fluidity of the membrane, primarily the length and saturation of fatty acid tails in the phospholipids.
-
Fatty Acid Tail Length: Shorter fatty acid tails result in increased fluidity because they pack together less tightly. Longer tails lead to decreased fluidity due to stronger hydrophobic interactions.
-
Fatty Acid Saturation: Unsaturated fatty acids (containing double bonds) create kinks in the tails, preventing tight packing and increasing fluidity. Saturated fatty acids (no double bonds) pack tightly, reducing membrane fluidity.
-
Cholesterol: Cholesterol, an important component of animal cell membranes, acts as a fluidity buffer. At high temperatures, it reduces membrane fluidity by restricting phospholipid movement. At low temperatures, it prevents the membrane from solidifying by interfering with the tight packing of fatty acid tails.
-
The "Mosaic" Aspect: A Diverse Collection of Proteins
The mosaic aspect of the cell membrane refers to the diverse array of proteins embedded within the lipid bilayer. These proteins are not randomly distributed; their placement and orientation are crucial for specific functions. They are categorized into two main types:
Integral Membrane Proteins
These proteins are permanently embedded within the lipid bilayer, often spanning the entire width of the membrane (transmembrane proteins). They have hydrophobic regions that interact with the lipid tails and hydrophilic regions that interact with the aqueous environment on either side of the membrane.
- Functions of Integral Membrane Proteins: These proteins perform a wide variety of functions, including:
- Transport: Facilitating the movement of specific molecules across the membrane (e.g., ion channels, carrier proteins).
- Receptors: Binding to signaling molecules and initiating intracellular responses.
- Enzymes: Catalyzing biochemical reactions within the membrane.
- Anchors: Connecting the cytoskeleton to the extracellular matrix, maintaining cell shape and structure.
Peripheral Membrane Proteins
These proteins are not embedded within the lipid bilayer; instead, they are loosely associated with the membrane surface, often bound to integral membrane proteins or the polar head groups of phospholipids.
- Functions of Peripheral Membrane Proteins: These proteins are involved in a range of cellular processes, including:
- Enzymatic activity: Catalyzing reactions at the membrane surface.
- Cell signaling: Acting as intermediaries in signal transduction pathways.
- Structural support: Maintaining the integrity and shape of the cell.
Carbohydrates: The Glycalyx and Cell Recognition
Beyond lipids and proteins, carbohydrates play a crucial role in the cell membrane's structure and function. These carbohydrates are often attached to lipids (glycolipids) or proteins (glycoproteins), forming a carbohydrate-rich layer on the cell surface called the glycocalyx.
The Role of the Glycocalyx
The glycocalyx contributes significantly to the cell membrane's overall mosaic nature. Its functions include:
- Cell recognition and adhesion: The diverse array of carbohydrate structures on the glycocalyx allows for specific cell-cell recognition and adhesion, essential for tissue formation and immune responses.
- Protection: The glycocalyx acts as a protective barrier, shielding the cell from mechanical damage and pathogens.
- Lubrication: The carbohydrate layer reduces friction between cells, facilitating movement and preventing cell aggregation.
Dynamic Interactions: The Fluid Mosaic in Action
The fluid mosaic model isn't just a static description; it emphasizes the dynamic interactions between the membrane components. These interactions are essential for numerous cellular processes.
Membrane Fluidity and Function
Membrane fluidity is crucial for various cellular functions:
- Protein mobility: The lateral movement of proteins within the membrane allows for interactions between different proteins, facilitating signal transduction and other cellular processes.
- Membrane fusion and fission: The fluidity of the membrane allows for the fusion and fission of vesicles with the plasma membrane, essential for endocytosis and exocytosis.
- Cell growth and division: The dynamic nature of the membrane is crucial for cell growth, division, and shape changes.
Membrane Asymmetry and Function
The asymmetrical distribution of lipids and proteins between the two leaflets of the bilayer is also vital:
- Signal transduction: Specific lipid and protein compositions in the outer leaflet allow for specific interactions with extracellular signaling molecules.
- Membrane trafficking: The asymmetry of the membrane guides the transport of vesicles and proteins to their appropriate cellular locations.
Implications of Membrane Fluidity and Mosaicism
The fluid mosaic nature of the cell membrane has profound implications for cell biology and medicine:
- Drug delivery: Understanding membrane fluidity and protein structure is crucial for designing drugs that can effectively cross the cell membrane and reach their target sites.
- Disease pathogenesis: Changes in membrane fluidity or composition can lead to various diseases, including cancer, neurodegenerative disorders, and infectious diseases.
- Biotechnology: Manipulating membrane properties is crucial for biotechnological applications such as cell engineering and tissue regeneration.
Conclusion: A Dynamic and Versatile Structure
The "fluid mosaic model" is a powerful and accurate description of the cell membrane's intricate structure and function. Its fluidity, enabled by the dynamic lipid bilayer, and its mosaic composition, defined by the diverse array of embedded proteins and carbohydrates, create a dynamic and versatile structure essential for life. Understanding the dynamic interplay of these components is crucial to unraveling the complexities of cellular biology and developing new therapeutic strategies for various diseases. The ever-evolving research in this area continues to deepen our appreciation of the cell membrane’s remarkable abilities and its critical role in maintaining life's intricate processes. Further research into the specific interactions and functionalities of the components will continue to refine our understanding of this essential cellular component.
Latest Posts
Latest Posts
-
Two Lines That Intersect To Form Right Angles
Apr 19, 2025
-
What Type Of Reaction Releases Energy
Apr 19, 2025
-
Find The Geometric Mean Of 275 And 11
Apr 19, 2025
-
Which Of The Following Is The Quadratic Parent Function
Apr 19, 2025
-
3 X Square Root Of 3
Apr 19, 2025
Related Post
Thank you for visiting our website which covers about Why Is A Cell Membrane Called A Fluid Mosaic . We hope the information provided has been useful to you. Feel free to contact us if you have any questions or need further assistance. See you next time and don't miss to bookmark.