Why Does A Purine Always Pair With A Pyrimidine
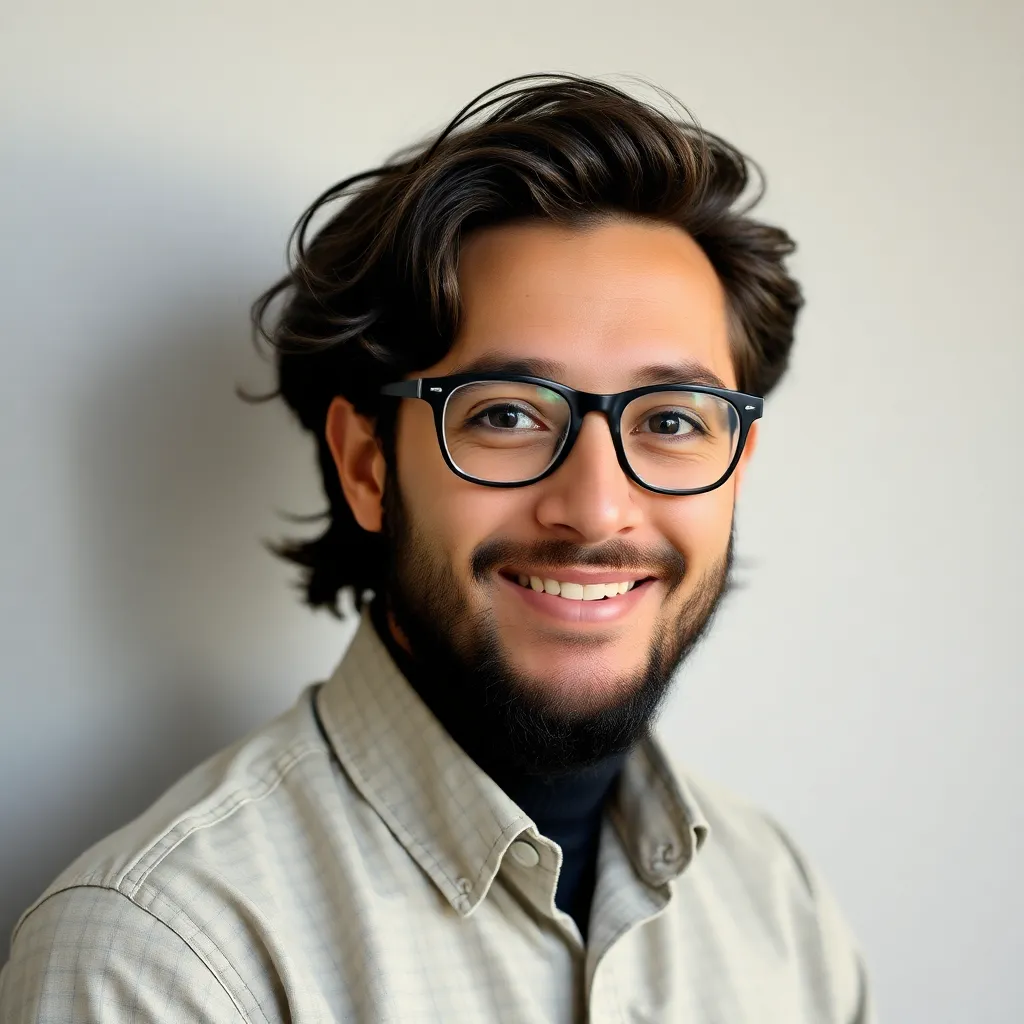
listenit
Apr 09, 2025 · 5 min read

Table of Contents
Why Does a Purine Always Pair with a Pyrimidine? Understanding Watson-Crick Base Pairing in DNA
The double helix structure of DNA, a cornerstone of molecular biology, relies fundamentally on the specific pairing of purine and pyrimidine bases. This precise pairing, known as Watson-Crick base pairing, is crucial for DNA replication, transcription, and the overall stability of the genetic material. But why does this specific pairing – a purine always with a pyrimidine – occur? The answer lies in a combination of factors related to molecular geometry, hydrogen bonding, and the overall energy minimization within the DNA double helix.
The Players: Purines and Pyrimidines
Before delving into the reasons behind the pairing, let's briefly revisit the molecular structures of the bases involved. DNA is composed of four nitrogenous bases: two purines – adenine (A) and guanine (G) – and two pyrimidines – cytosine (C) and thymine (T).
-
Purines: These are larger, double-ringed structures. Adenine and guanine both possess a six-membered ring fused to a five-membered ring. This larger size influences their pairing potential.
-
Pyrimidines: These are smaller, single-ringed structures. Cytosine and thymine consist of only a single six-membered ring. This smaller size is critical for maintaining the uniform diameter of the DNA helix.
The Key: Hydrogen Bonding and Molecular Geometry
The fundamental reason a purine always pairs with a pyrimidine is the requirement for maintaining a constant diameter of the DNA double helix. If two purines were to pair (e.g., A-A or G-G), the double helix would bulge outward. Conversely, if two pyrimidines were to pair (e.g., C-C or T-T), the helix would constrict. Both scenarios disrupt the stable, uniform structure crucial for DNA function.
The specific pairing is further reinforced by hydrogen bonding. Hydrogen bonds are weak, non-covalent bonds that form between a hydrogen atom and a highly electronegative atom like oxygen or nitrogen. These bonds are numerous and relatively weak individually, but collectively they provide significant stability to the DNA double helix.
-
Adenine (A) and Thymine (T): These form two hydrogen bonds. A specific hydrogen donor and acceptor on each base align perfectly to facilitate these bonds. Any other combination would lead to steric clashes and misalignment of the hydrogen bond donors and acceptors.
-
Guanine (G) and Cytosine (C): These form three hydrogen bonds. Again, the specific arrangement of hydrogen bond donors and acceptors allows for a perfect fit, optimizing bond strength and helix stability. The addition of a third hydrogen bond contributes to the greater stability of the G-C base pair compared to the A-T base pair.
Beyond Hydrogen Bonds: Other Factors Contributing to Specificity
While hydrogen bonding is the dominant factor, other forces also contribute to the purine-pyrimidine pairing specificity:
-
Hydrophobic Interactions: The hydrophobic (water-repelling) nature of the bases encourages them to stack together in the interior of the DNA double helix, away from the surrounding water molecules. This stacking interaction further stabilizes the structure.
-
Van der Waals Forces: These weak, short-range attractive forces between molecules also play a minor role in stabilizing base stacking and base pairing.
-
Steric Hindrance: The sizes and shapes of the bases are such that only the purine-pyrimidine pairings can avoid significant steric clashes (collisions between atoms). Attempts to pair two purines or two pyrimidines result in unfavorable steric interactions, destabilizing the DNA structure.
The Consequences of Incorrect Pairing
The precise purine-pyrimidine pairing is not just a structural quirk; it has profound implications for the cell:
-
DNA Replication: During DNA replication, the specific base pairing is essential for accurate duplication of the genetic material. DNA polymerase, the enzyme responsible for replication, utilizes the Watson-Crick base pairing rules to ensure that the newly synthesized strand is a faithful copy of the template strand. Incorrect pairing can lead to mutations, altering the genetic information and potentially having serious consequences.
-
Transcription: The transcription of DNA into RNA also relies on base pairing. RNA polymerase uses the DNA template strand to synthesize a complementary RNA molecule, following the same pairing rules (with uracil (U) substituting for thymine (T) in RNA). Mistakes in transcription can affect the production of proteins and have significant consequences.
-
DNA Repair Mechanisms: Cells have evolved sophisticated DNA repair mechanisms to correct errors in base pairing that may occur due to spontaneous mutations or environmental damage. These repair pathways rely on the ability to recognize and correct mismatched base pairs.
Evolutionary Significance of Base Pairing
The specific purine-pyrimidine base pairing is not an accident. It's a fundamental feature of life that has been conserved throughout evolution. This system's efficiency and accuracy in maintaining the integrity of genetic information are crucial for the survival and propagation of all living organisms. The system is robust, minimizing errors during replication and transcription, and possessing mechanisms to correct rare mismatches.
Applications and Further Research
Understanding the precise reasons behind purine-pyrimidine base pairing has far-reaching implications. This knowledge underpins many aspects of molecular biology and biotechnology:
-
Drug Design: Many drugs target DNA, interfering with DNA replication or transcription. Understanding base pairing is crucial for designing effective and specific drugs that can interact with DNA without causing widespread damage.
-
Genomic Sequencing: Accurate DNA sequencing relies heavily on the principle of base pairing. New sequencing technologies exploit this principle to determine the order of bases in a DNA molecule.
-
Gene Editing: CRISPR-Cas9 gene editing technologies leverage the base pairing principles to precisely target and modify specific genes.
Ongoing research continues to explore the nuances of base pairing, investigating factors influencing its fidelity and potential variations in different organisms. For instance, the discovery of unusual base pairings in certain contexts (e.g., during DNA repair) highlights the complexity and adaptability of this fundamental biological process. While the basic principle remains consistent, the subtle variations add layers of complexity and resilience to the genetic system.
Conclusion
The seemingly simple rule that a purine always pairs with a pyrimidine in DNA is far from trivial. It's a consequence of a complex interplay between molecular geometry, hydrogen bonding, hydrophobic interactions, and steric constraints, all working together to ensure the stability, accuracy, and fidelity of the genetic material. This elegant system underpins the very foundation of life, and its ongoing study continues to reveal deeper insights into the workings of the living cell. The precise pairing mechanism is not just a structural feature; it's a cornerstone of life itself, ensuring the accurate transmission of genetic information across generations and the survival of species.
Latest Posts
Latest Posts
-
How Do You Calculate Freezing Point
Apr 17, 2025
-
What Is The Formula For Magnesium Iodide
Apr 17, 2025
-
Name The Given Alkenes Using Systematic Names
Apr 17, 2025
-
How Are Isotopes Of The Same Element Alike
Apr 17, 2025
-
What Is 9 10 As A Percentage
Apr 17, 2025
Related Post
Thank you for visiting our website which covers about Why Does A Purine Always Pair With A Pyrimidine . We hope the information provided has been useful to you. Feel free to contact us if you have any questions or need further assistance. See you next time and don't miss to bookmark.