Which Functional Group Can Act As An Acid
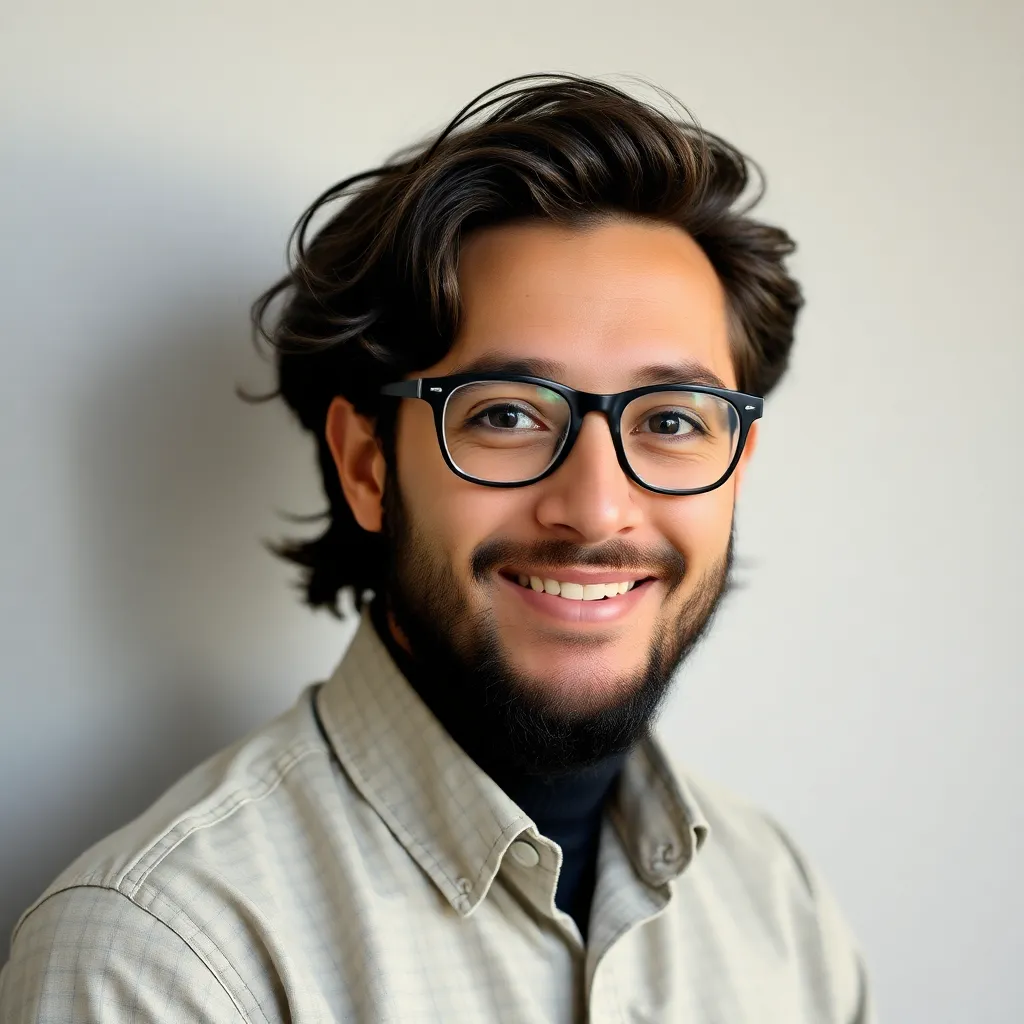
listenit
Apr 17, 2025 · 6 min read

Table of Contents
Which Functional Groups Can Act as Acids? A Comprehensive Guide
Understanding acidity in organic chemistry is crucial for predicting reactivity and designing chemical reactions. Many functional groups possess acidic protons that can be donated to a base. This article explores the various functional groups capable of acting as acids, focusing on their relative acidity, influencing factors, and examples.
Understanding Acidity: The Basics
Before delving into specific functional groups, let's review the fundamental concept of acidity. Acidity is a measure of a molecule's willingness to donate a proton (H⁺). A stronger acid readily donates its proton, while a weaker acid holds onto its proton more tightly. This ability is quantified by the acid dissociation constant (Ka), where a higher Ka value indicates a stronger acid. Alternatively, the pKa (=-log Ka) is commonly used, with a lower pKa representing a stronger acid.
The acidity of a molecule is primarily determined by the stability of its conjugate base after proton donation. A more stable conjugate base leads to a stronger acid. Factors influencing conjugate base stability include:
- Electronegativity: More electronegative atoms stabilize negative charge more effectively.
- Resonance: Delocalization of the negative charge through resonance structures significantly enhances stability.
- Inductive Effects: Electron-withdrawing groups stabilize negative charges through inductive effects.
- Hybridization: The more s-character in the hybridized orbital holding the negative charge, the closer the electrons are to the nucleus, leading to greater stability.
Functional Groups Exhibiting Acidity
Numerous functional groups can act as acids, differing in their strength and underlying reasons. Let's examine the most prevalent ones:
1. Carboxylic Acids (RCOOH)
Carboxylic acids are arguably the most common and strongest organic acids. The acidity stems from the carboxylate anion (RCOO⁻) formed after proton donation. This anion is exceptionally stable due to two significant factors:
- Resonance Stabilization: The negative charge is delocalized between the two oxygen atoms through resonance, distributing the charge and reducing its overall density. This resonance stabilization significantly lowers the energy of the conjugate base.
- Electronegativity of Oxygen: The oxygen atoms are highly electronegative, effectively accommodating the negative charge.
Example: Acetic acid (CH₃COOH), a weak acid, has a pKa of approximately 4.76. The highly stable acetate ion (CH₃COO⁻) makes acetic acid a relatively strong acid compared to many other organic compounds.
2. Phenols (ArOH)
Phenols are aromatic compounds with a hydroxyl group (-OH) directly attached to the aromatic ring. Phenols are weaker acids than carboxylic acids but stronger than alcohols. Their acidity is attributed to:
- Resonance Stabilization: The negative charge on the phenoxide ion (ArO⁻) is delocalized across the aromatic ring through resonance, stabilizing the conjugate base. The extent of resonance stabilization depends on the substituents on the aromatic ring. Electron-withdrawing groups increase acidity, while electron-donating groups decrease it.
- Inductive Effect: The aromatic ring's electron-withdrawing inductive effect also contributes to the stabilization of the phenoxide ion.
Example: Phenol (C₆H₅OH) has a pKa of around 10, indicating it's a weaker acid than carboxylic acids but stronger than alcohols.
3. Alcohols (ROH)
Alcohols are considerably weaker acids than carboxylic acids and phenols. Their acidity is mainly influenced by:
- Electronegativity of Oxygen: The oxygen atom's electronegativity helps stabilize the alkoxide ion (RO⁻) formed after proton donation. However, this stabilization is less effective compared to the resonance stabilization in carboxylates and phenoxides.
- Inductive Effects: Electron-withdrawing groups near the hydroxyl group can increase the acidity of alcohols by stabilizing the alkoxide ion through inductive effects.
Example: Methanol (CH₃OH) has a high pKa of around 15.7, indicating its weak acidity.
4. Thiols (RSH)
Thiols, also known as mercaptans, contain a sulfhydryl group (-SH). They are significantly more acidic than alcohols due to the larger size and lower electronegativity of sulfur compared to oxygen. The larger size of sulfur allows for better dispersal of the negative charge in the thiolate ion (RS⁻). The lower electronegativity of sulfur makes it less effective at stabilizing negative charge directly, but the better dispersal outweighs this effect.
Example: Ethanethiol (CH₃CH₂SH) has a pKa of around 10.6, showing its greater acidity compared to ethanol.
5. β-Dicarbonyl Compounds
β-Dicarbonyl compounds possess two carbonyl groups separated by a methylene group (-CH₂-). The acidity of these compounds is remarkably high due to the exceptional stability of their conjugate bases:
- Resonance Stabilization: The conjugate base is stabilized by two resonance structures, each with a negative charge delocalized across two oxygen atoms. This extensive delocalization leads to high stability.
- Inductive Effects: The two carbonyl groups exert strong electron-withdrawing inductive effects, further stabilizing the negative charge.
Example: Acetylacetone (CH₃COCH₂COCH₃) has a pKa of around 9, reflecting its relatively high acidity.
6. Terminal Alkynes (RC≡CH)
Terminal alkynes possess a hydrogen atom directly bonded to a sp-hybridized carbon atom. This hydrogen is surprisingly acidic. Acidity arises from:
- High s-character of the sp orbital: The sp hybridized carbon atom has 50% s-character, pulling electron density towards itself and stabilizing the negative charge on the conjugate base (RC≡C⁻). The increased s-character places the electron pair closer to the nucleus, leading to greater stability.
Example: Ethyne (HC≡CH) has a pKa of around 25, much higher than alcohols but significantly lower than alkanes.
Factors Affecting Acidity Within Functional Groups
Beyond the inherent properties of functional groups, several factors can modulate their acidity:
- Inductive Effects of Substituents: Electron-withdrawing groups (-NO₂, -Cl, -F) increase acidity by stabilizing the conjugate base through inductive effects. Conversely, electron-donating groups (-CH₃, -OCH₃) decrease acidity.
- Steric Hindrance: Bulky substituents can hinder the approach of a base, decreasing the rate of proton donation but not necessarily altering the equilibrium acidity.
- Solvent Effects: The solvent can significantly affect the acidity of a compound by influencing the stabilization of the conjugate base. Protic solvents like water can stabilize the conjugate base through hydrogen bonding, increasing the apparent acidity.
- Resonance Effects of Substituents: Substituents on the aromatic ring in phenols or the carbon chain near the acidic functional group will affect resonance stabilization of the conjugate base.
Conclusion
Many functional groups can act as acids, varying widely in their strength. Understanding the factors influencing acidity—resonance, inductive effects, electronegativity, and hybridization—is critical to predicting the reactivity of organic molecules. The relative acidity of these groups (from strongest to weakest) roughly follows the order: carboxylic acids > phenols > thiols > β-dicarbonyl compounds > terminal alkynes > alcohols. However, specific substituents and environmental factors can significantly alter this order in individual cases. A thorough grasp of these concepts is essential for success in organic chemistry. Remember to consider the overall context of a molecule’s structure and surroundings when predicting its acidity.
Latest Posts
Latest Posts
-
How To Convert Mmhg To Pa
Apr 19, 2025
-
Convert From Rectangular To Polar Coordinates
Apr 19, 2025
-
What Is The Lcm Of 4 And 14
Apr 19, 2025
-
Which Quantities Are Conserved In All Chemical Reactions
Apr 19, 2025
-
How Many Atoms Are In 3 5 Moles Of Arsenic Atoms
Apr 19, 2025
Related Post
Thank you for visiting our website which covers about Which Functional Group Can Act As An Acid . We hope the information provided has been useful to you. Feel free to contact us if you have any questions or need further assistance. See you next time and don't miss to bookmark.