Where Is The Chemical Energy Stored In Atp
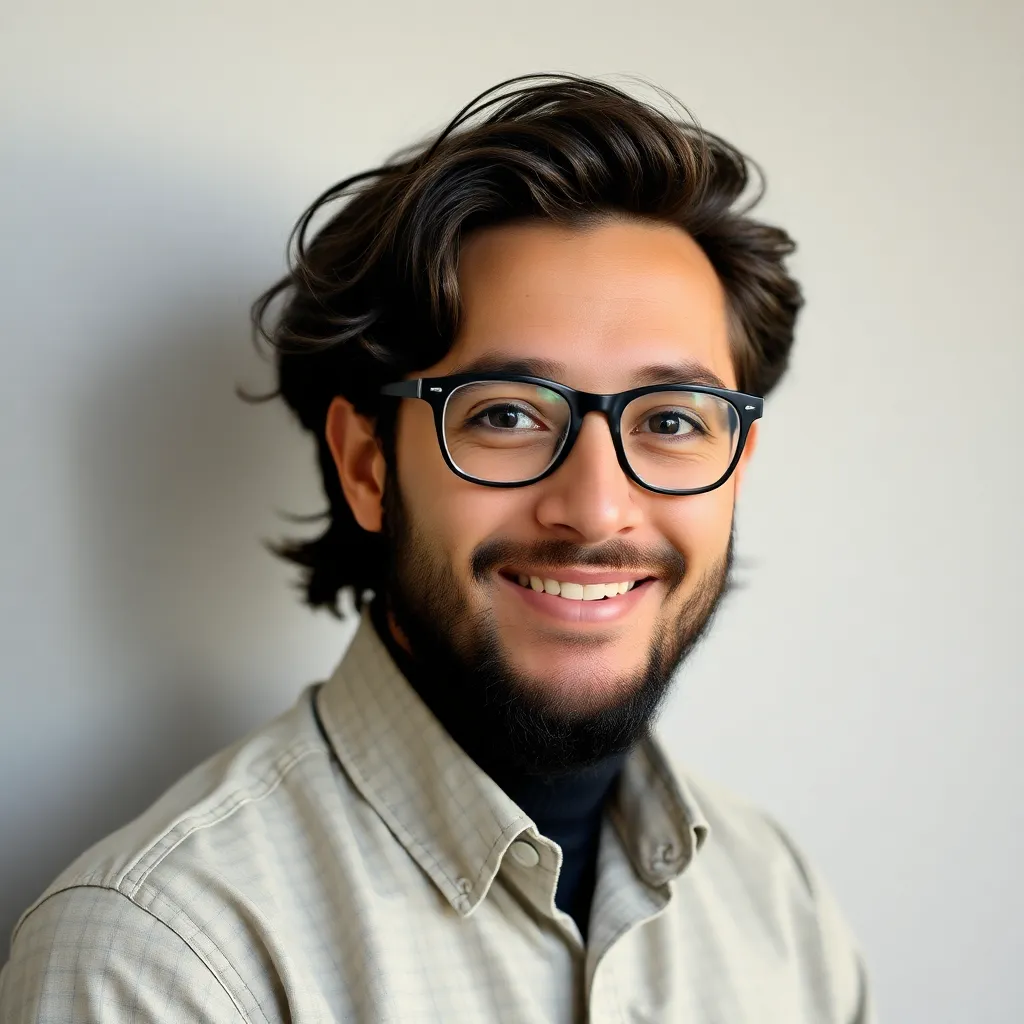
listenit
Apr 17, 2025 · 5 min read

Table of Contents
Where is the Chemical Energy Stored in ATP? Unraveling the Powerhouse of the Cell
Adenosine triphosphate (ATP) is the fundamental energy currency of all living cells. Its crucial role in powering countless cellular processes, from muscle contraction to protein synthesis, makes understanding its energy storage mechanism paramount. But where exactly is this chemical energy stored within the ATP molecule? This article delves deep into the intricate structure of ATP and explains the precise location and nature of its stored energy.
The Structure of ATP: A Molecular Powerhouse
Before exploring energy storage, let's examine ATP's molecular architecture. ATP is a nucleotide composed of three main components:
- Adenine: A nitrogenous base with a complex ring structure.
- Ribose: A five-carbon sugar molecule that forms the backbone of the ATP molecule.
- Triphosphate group: A chain of three phosphate groups (PO<sub>4</sub><sup>3−</sup>) linked together. This is where the action—and the energy—happens.
The three phosphate groups are linked via high-energy phosphoanhydride bonds. These are not ordinary chemical bonds; they are characterized by a high amount of potential energy due to the strong electrostatic repulsion between the negatively charged phosphate groups. It is this repulsion that is key to understanding ATP's energy storage capacity.
High-Energy Phosphoanhydride Bonds: The Key to Energy Storage
The key to understanding ATP's energy storage lies within the phosphoanhydride bonds connecting the phosphate groups. These bonds are high-energy because of several factors:
-
Electrostatic repulsion: The negatively charged phosphate groups strongly repel each other. This repulsion creates an unstable state, storing considerable potential energy. Imagine compressing a spring; the more you compress it, the more potential energy it stores. Similarly, the tightly packed phosphate groups in ATP store substantial potential energy due to their mutual repulsion.
-
Resonance stabilization: The phosphate groups exhibit resonance stabilization when they are separated. In other words, when a phosphate group is released, the resulting molecules are more stable than the original ATP molecule. This increased stability releases energy.
-
Hydration: The products of ATP hydrolysis (ADP and inorganic phosphate) are more hydrated than ATP itself. This hydration releases energy, further contributing to the overall energy yield.
Hydrolysis of ATP: Releasing the Stored Energy
The energy stored in ATP is released through a process called hydrolysis. Hydrolysis involves the breaking of a phosphoanhydride bond using a water molecule. Typically, the bond between the second and third phosphate groups is broken, yielding:
- Adenosine diphosphate (ADP): ATP minus one phosphate group.
- Inorganic phosphate (Pi): The released phosphate group.
- Energy: Released in the form of usable energy for cellular processes.
This hydrolysis reaction is highly exergonic, meaning it releases a significant amount of free energy (approximately -7.3 kcal/mol under standard conditions). This released energy is then coupled to endergonic reactions—reactions that require energy input—making them thermodynamically favorable.
Coupling ATP Hydrolysis to Endergonic Reactions
Many cellular processes require energy input to proceed. These are called endergonic reactions, and they would not occur spontaneously without an energy source. Cells cleverly utilize the energy released from ATP hydrolysis to drive these endergonic reactions. This is called energy coupling.
A prime example is the synthesis of a protein molecule. The formation of peptide bonds between amino acids is an endergonic process. However, by coupling this reaction to the exergonic hydrolysis of ATP, the overall reaction becomes thermodynamically favorable, allowing the protein synthesis to proceed. Essentially, the energy released from ATP hydrolysis fuels the endergonic protein synthesis.
Beyond ATP Hydrolysis: Other Mechanisms of Energy Release
While ATP hydrolysis is the predominant method of energy release, other mechanisms also contribute to ATP's energy-providing capabilities:
-
Phosphorylation: The transfer of a phosphate group from ATP to another molecule (phosphorylation) can activate that molecule or change its shape. This can be crucial in regulating enzyme activity or driving conformational changes necessary for cellular processes. Kinases, a vital class of enzymes, catalyze these phosphorylation reactions.
-
Substrate-level phosphorylation: This is a mechanism of ATP synthesis where a phosphate group is directly transferred from a high-energy phosphorylated substrate molecule to ADP, forming ATP. This occurs during glycolysis and the citric acid cycle.
The Importance of ATP in Cellular Processes: A Broad Overview
The ubiquitous role of ATP in cellular processes underscores its significance as the fundamental energy currency. Here are just a few examples:
-
Muscle contraction: ATP provides the energy needed for the interaction between actin and myosin filaments, causing muscle fibers to shorten and generate force.
-
Active transport: Moving molecules against their concentration gradients (active transport) requires energy from ATP. Sodium-potassium pumps, for example, utilize ATP to maintain cellular ion balance.
-
Biosynthesis: The synthesis of complex molecules like proteins, carbohydrates, and lipids requires ATP as an energy source.
-
Nerve impulse transmission: The propagation of nerve impulses depends on ATP-driven ion pumps maintaining the electrochemical gradients across neuronal membranes.
-
Cell division: The complex processes involved in cell division, including DNA replication and chromosome segregation, are all heavily reliant on ATP.
ATP Regeneration: The Continuous Cycle of Energy
ATP is constantly being used and regenerated within the cell. The continuous cycle of ATP hydrolysis and regeneration is crucial for maintaining a steady supply of energy to power cellular activities. The primary mechanisms of ATP regeneration include:
-
Cellular respiration: This is the most significant pathway for ATP regeneration in aerobic organisms. It involves the breakdown of glucose in the presence of oxygen, generating a large amount of ATP through oxidative phosphorylation.
-
Photosynthesis: In plants and other photosynthetic organisms, light energy is used to drive ATP synthesis.
-
Fermentation: This anaerobic pathway generates a smaller amount of ATP compared to cellular respiration, but it's essential for survival in oxygen-deficient environments.
Conclusion: Understanding ATP's Energy Storage is Crucial
The energy stored in ATP isn't located in a single, easily definable spot within the molecule; rather, it's an emergent property of the entire structure. The high-energy phosphoanhydride bonds, arising from electrostatic repulsion, resonance stabilization, and hydration changes, are the primary storage sites. The release of this stored energy through hydrolysis powers an astonishing array of cellular processes, underpinning the very essence of life. Understanding the precise mechanism of ATP's energy storage and its role in cellular functions is crucial for comprehending the fundamental principles of biology and biochemistry. Further research continues to unveil the intricate details of ATP's role in various biological phenomena, promising to reveal even more about the intricate machinery of life itself.
Latest Posts
Latest Posts
-
Equation Of A Circle In The Xy Plane
Apr 19, 2025
-
How To Convert Mmhg To Pa
Apr 19, 2025
-
Convert From Rectangular To Polar Coordinates
Apr 19, 2025
-
What Is The Lcm Of 4 And 14
Apr 19, 2025
-
Which Quantities Are Conserved In All Chemical Reactions
Apr 19, 2025
Related Post
Thank you for visiting our website which covers about Where Is The Chemical Energy Stored In Atp . We hope the information provided has been useful to you. Feel free to contact us if you have any questions or need further assistance. See you next time and don't miss to bookmark.