What Type Of Conduction Takes Place In Unmyelinated Axons
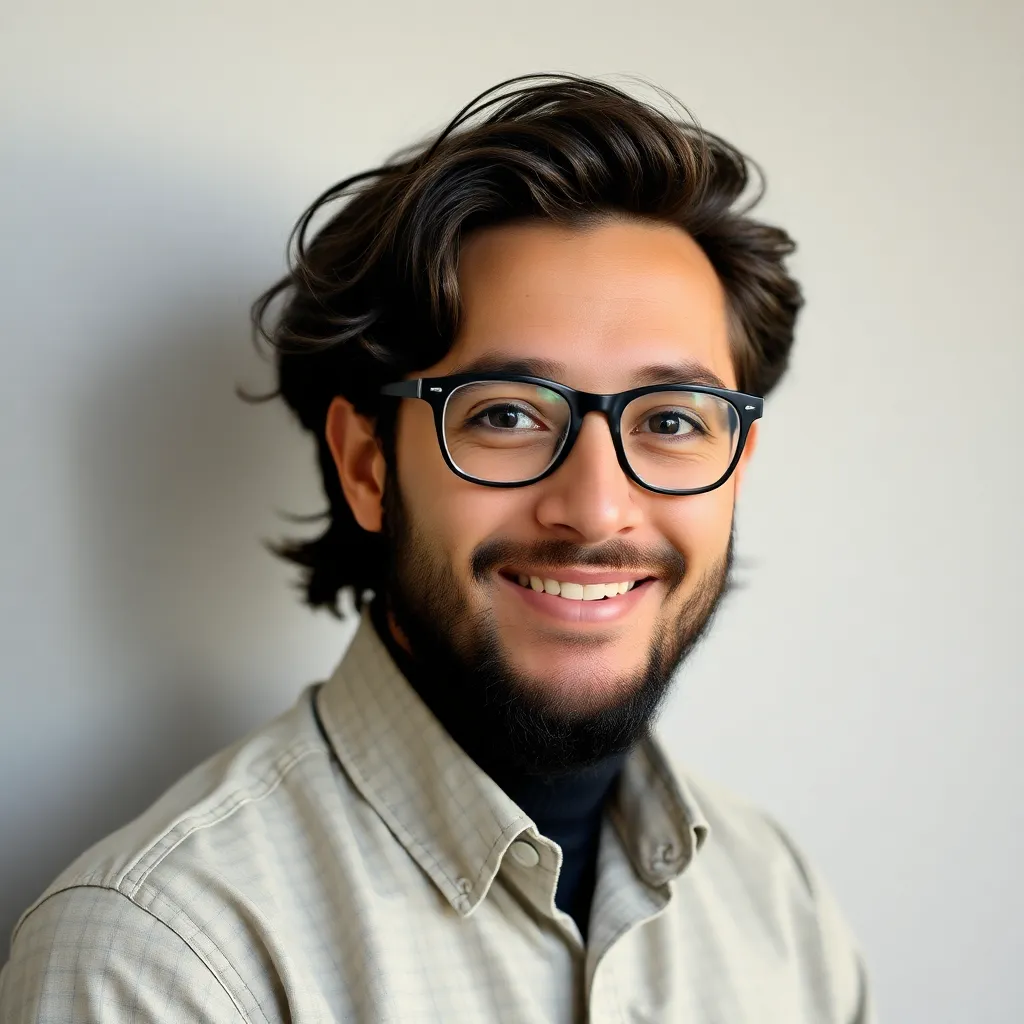
listenit
May 27, 2025 · 5 min read
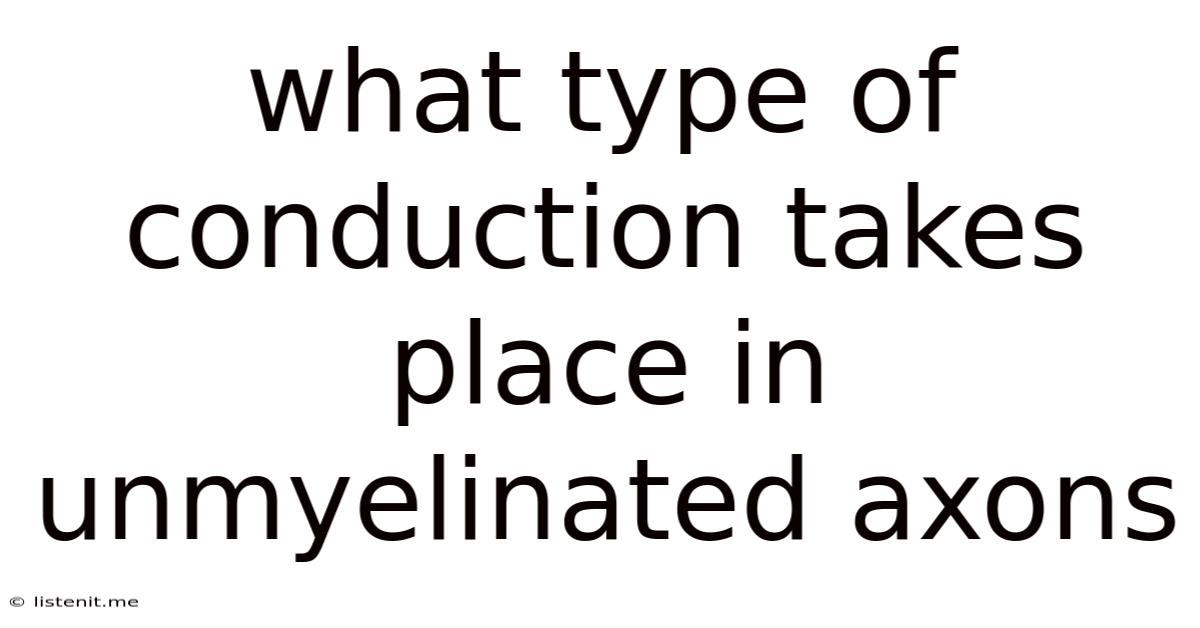
Table of Contents
What Type of Conduction Takes Place in Unmyelinated Axons?
Understanding how nerve impulses travel along axons is fundamental to comprehending the nervous system's function. Axons, the long projections of nerve cells, transmit signals via electrical impulses. However, the mechanism of this transmission differs significantly depending on whether the axon is myelinated or unmyelinated. This article delves deep into the type of conduction that occurs in unmyelinated axons, exploring its intricacies and contrasting it with the conduction process in myelinated axons.
Conduction in Unmyelinated Axons: Continuous Conduction
Unlike myelinated axons, which exhibit saltatory conduction, unmyelinated axons employ continuous conduction. This means the action potential propagates along the entire length of the axon membrane without interruption. The impulse travels as a wave of depolarization, sequentially activating adjacent segments of the axon membrane. This process is slower and less energy-efficient than saltatory conduction.
The Step-by-Step Process of Continuous Conduction
-
Depolarization: The process begins when a stimulus reaches the axon hillock, the region where the axon originates from the cell body. If the stimulus is strong enough to reach the threshold potential, voltage-gated sodium (Na+) channels open. This allows an influx of Na+ ions into the axon, causing a rapid depolarization of the membrane. The inside of the axon becomes positively charged relative to the outside.
-
Action Potential Generation: This depolarization triggers an action potential, a brief but significant change in the membrane potential. The action potential is an all-or-none event; once the threshold is reached, the action potential proceeds to completion.
-
Propagation: The depolarization spreads passively to the adjacent membrane segments. This passive spread is due to the local current flow generated by the initial depolarization. The positively charged ions inside the axon move along the axon, depolarizing the neighboring areas.
-
Sodium Channel Activation in Adjacent Segments: As the depolarization reaches neighboring segments, the voltage-gated Na+ channels in these segments open, leading to further influx of Na+ ions and depolarization of those segments. This process repeats itself along the entire length of the axon.
-
Repolarization: Following depolarization, voltage-gated potassium (K+) channels open. This allows an outflow of K+ ions, restoring the resting membrane potential. This repolarization phase ensures that the action potential propagates in only one direction. The refractory period prevents the action potential from traveling backward.
-
Sequential Activation: This sequential activation of voltage-gated ion channels and the resulting depolarization-repolarization cycle propagate the action potential along the axon. Because each segment must be individually depolarized and repolarized, this process is relatively slow.
Comparison with Saltatory Conduction in Myelinated Axons
It's crucial to understand the differences between continuous conduction in unmyelinated axons and saltatory conduction in myelinated axons. Saltatory conduction, meaning "leaping," is significantly faster. Myelin, a fatty insulating sheath produced by glial cells (oligodendrocytes in the central nervous system and Schwann cells in the peripheral nervous system), wraps around the axon, creating gaps called Nodes of Ranvier.
In saltatory conduction, the action potential "jumps" from one Node of Ranvier to the next. The myelin sheath prevents ion flow except at the Nodes, where the concentration of voltage-gated ion channels is high. This "jumping" mechanism significantly increases the speed of conduction.
Here's a table summarizing the key differences:
Feature | Continuous Conduction (Unmyelinated) | Saltatory Conduction (Myelinated) |
---|---|---|
Speed | Slow | Fast |
Myelin | Absent | Present |
Propagation | Along the entire axon membrane | From Node of Ranvier to Node of Ranvier |
Energy Cost | High | Low |
Axon Diameter | Often smaller | Often larger |
Factors Influencing Conduction Speed in Unmyelinated Axons
Several factors influence the speed of continuous conduction in unmyelinated axons:
-
Axon Diameter: Larger diameter axons conduct impulses faster. A larger diameter reduces the internal resistance to current flow, enabling faster propagation of the action potential.
-
Temperature: Higher temperatures generally lead to faster conduction speeds because increased temperature accelerates ion movement across the membrane.
-
Membrane Properties: The specific properties of the axon membrane, such as the density and types of ion channels, can influence the speed of conduction.
-
Axon Length: Longer axons naturally take longer for the impulse to travel the full length, although this is less significant compared to the impact of diameter.
The Role of Ion Channels in Continuous Conduction
The precise functioning of voltage-gated Na+ and K+ channels is paramount for continuous conduction. Their opening and closing are tightly regulated by changes in membrane potential. Any disruption to these channels can severely impair or completely block impulse transmission. This explains why certain toxins that target ion channels can have devastating neurological effects.
Clinical Significance of Understanding Conduction in Unmyelinated Axons
Understanding the mechanisms of continuous conduction is crucial in various clinical contexts:
-
Neurological Disorders: Diseases affecting the myelin sheath, such as multiple sclerosis, can disrupt saltatory conduction. However, understanding unmyelinated axon conduction provides a baseline for comparing nerve function in the presence or absence of myelin.
-
Drug Development: Drugs targeting ion channels often have specific effects on nerve conduction. Understanding the differences in conduction mechanisms in myelinated and unmyelinated axons is vital for designing drugs with targeted effects.
-
Pain Management: Many pain fibers are unmyelinated C-fibers. Understanding the specifics of their conduction helps develop effective pain management strategies.
-
Neuropathies: Damage to peripheral nerves affecting both myelinated and unmyelinated fibers can be analyzed based on the differences in conduction velocity. This is useful for diagnosis.
Conclusion: Continuous Conduction - A Fundamental Aspect of Neural Function
Continuous conduction in unmyelinated axons, while slower than saltatory conduction, is a vital process for transmitting nerve impulses. This mechanism, dependent on the sequential activation of voltage-gated ion channels along the axon's membrane, underlines the intricacies of neural communication. Understanding its nuances is fundamental to appreciating the complexity of the nervous system and its susceptibility to various diseases and conditions. Further research continues to unveil finer details about the intricacies of ion channel kinetics and their role in the precise regulation of nerve impulse transmission along unmyelinated axons. This deeper understanding is instrumental in advancing our knowledge of neurological function and the development of novel therapeutic strategies. The efficiency and speed of continuous conduction, although slower than saltatory conduction, are crucial for a variety of physiological processes. Further research, using advanced technologies such as patch clamping and advanced imaging, will continue to elucidate the finer mechanisms and potential therapeutic targets within this fundamental process of neuronal signalling.
Latest Posts
Latest Posts
-
According To Sutherlands Differential Association Theory
May 28, 2025
-
Which Risks Are Direct Results Of Implementing Risk Responses
May 28, 2025
-
Which Of These Constitutes The Pectoral Girdle
May 28, 2025
-
Radiation Oncology Case Rate Value Based Payment Program Act
May 28, 2025
-
Which Of The Following Is Predominantly Made Up Of Myosin
May 28, 2025
Related Post
Thank you for visiting our website which covers about What Type Of Conduction Takes Place In Unmyelinated Axons . We hope the information provided has been useful to you. Feel free to contact us if you have any questions or need further assistance. See you next time and don't miss to bookmark.