What Is The Monomer Used To Make Protein
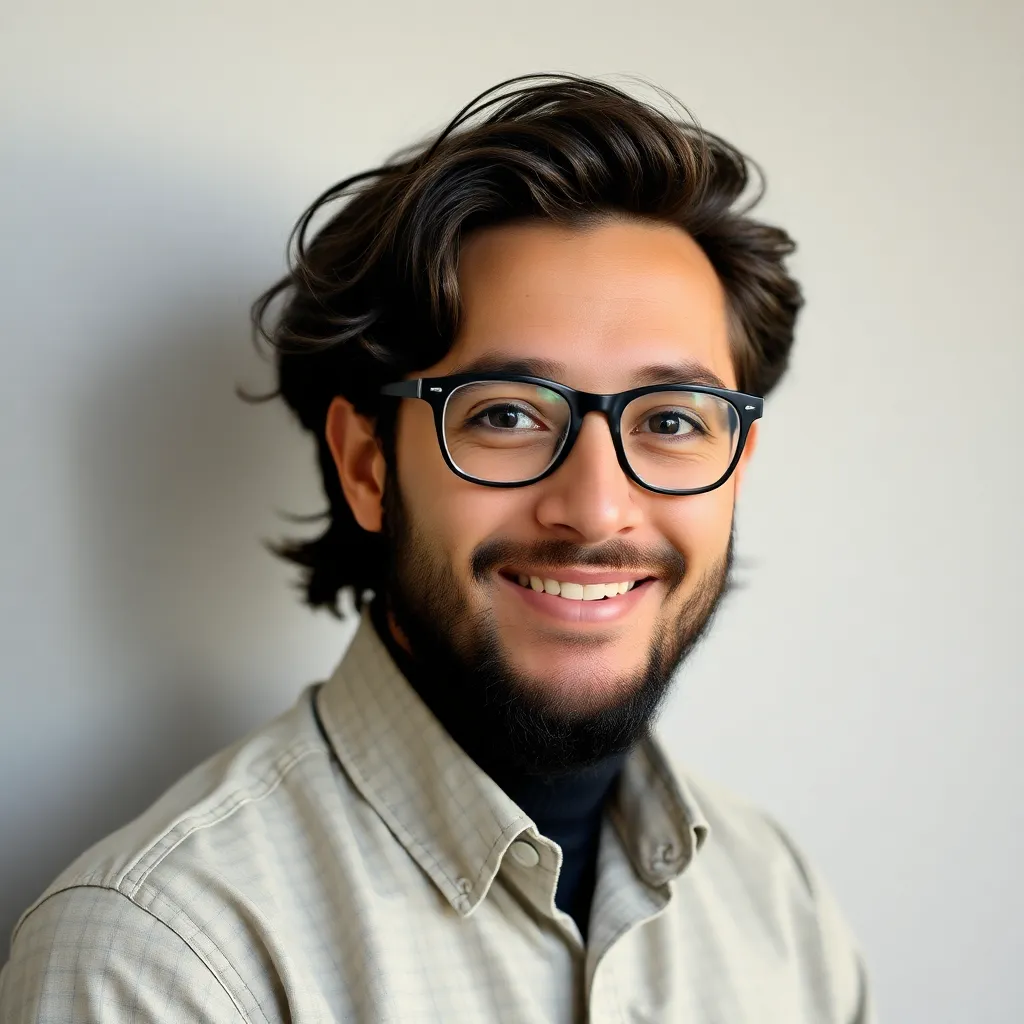
listenit
May 13, 2025 · 6 min read
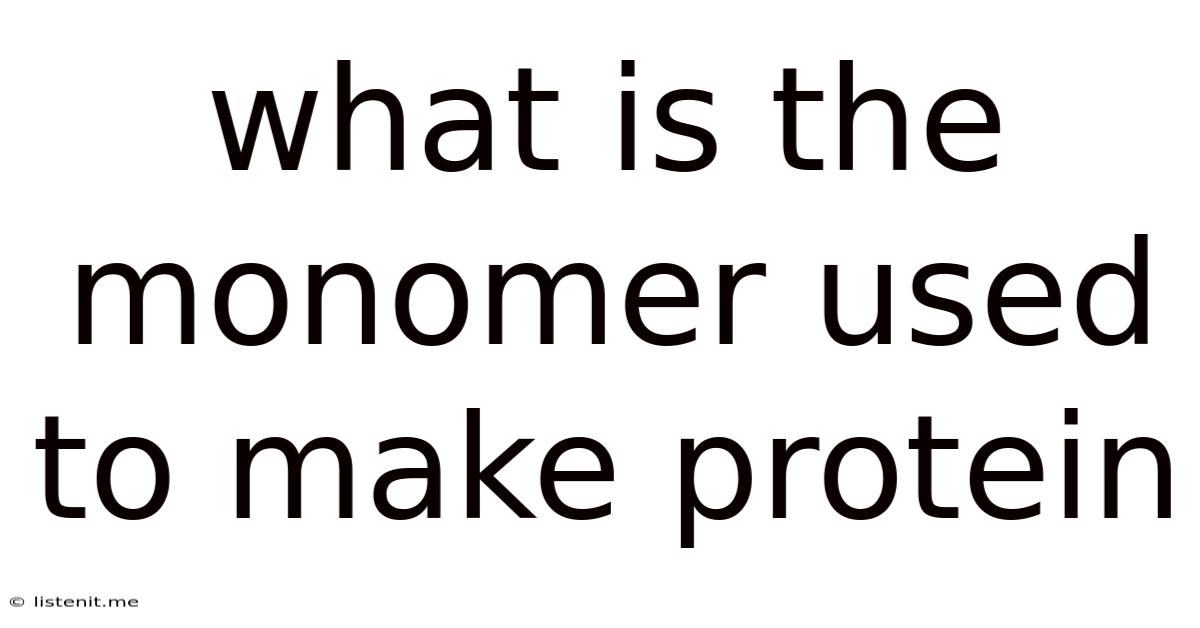
Table of Contents
What is the Monomer Used to Make Protein?
Proteins are the workhorses of the cell, performing a vast array of crucial functions. From catalyzing biochemical reactions as enzymes to providing structural support as collagen, proteins are essential for life as we know it. But what are these complex molecules actually made of? The answer lies in their fundamental building blocks: amino acids, the monomers that combine to create the diverse world of proteins.
Understanding Amino Acids: The Building Blocks of Proteins
Amino acids are organic molecules containing a central carbon atom (the alpha carbon) bonded to four different chemical groups:
- An amino group (-NH₂): This group is basic and gives amino acids their name.
- A carboxyl group (-COOH): This group is acidic and plays a crucial role in peptide bond formation.
- A hydrogen atom (-H): A simple hydrogen atom attached to the alpha carbon.
- A variable side chain (R-group): This is the unique part of each amino acid, determining its properties and characteristics. The R-group can be as simple as a hydrogen atom (as in glycine) or a complex structure containing rings, sulfur atoms, or charged groups.
It's this variable R-group that distinguishes the 20 standard amino acids used by living organisms to synthesize proteins. These 20 amino acids, each with their unique chemical properties, are the alphabet of the protein language, combining in different sequences to form countless protein structures with diverse functions.
The Diversity of Amino Acid R-Groups
The R-groups of amino acids dictate their properties, which are broadly categorized as:
-
Nonpolar (hydrophobic): These R-groups are largely composed of carbon and hydrogen atoms, repelling water. Examples include alanine, valine, leucine, isoleucine, methionine, phenylalanine, tryptophan, and proline. They often cluster together in the interior of proteins, away from the aqueous environment.
-
Polar (hydrophilic): These R-groups contain oxygen, nitrogen, or sulfur atoms, making them more attracted to water. Examples include serine, threonine, cysteine, asparagine, glutamine, tyrosine. They frequently appear on the surface of proteins, interacting with the surrounding water molecules.
-
Charged (hydrophilic): These R-groups carry a net positive or negative charge at physiological pH. Examples include:
- Acidic (negatively charged): Aspartic acid and glutamic acid.
- Basic (positively charged): Lysine, arginine, and histidine. These charged amino acids contribute to protein-protein interactions and enzyme activity.
Peptide Bond Formation: Linking Amino Acids
Amino acids are linked together through a process called peptide bond formation. This occurs between the carboxyl group (-COOH) of one amino acid and the amino group (-NH₂) of another amino acid. A molecule of water is removed during this condensation reaction, resulting in a covalent bond between the two amino acids, forming a dipeptide.
This process can be repeated numerous times, adding amino acids one by one to create a polypeptide chain. The sequence of amino acids in this chain determines the protein's primary structure, which dictates its higher-order structure and ultimately its function.
The peptide bond itself possesses partial double-bond character, resulting in a rigid, planar structure. This structural rigidity influences the overall folding and conformation of the protein.
From Monomers to Macromolecules: Protein Structure and Function
The linear sequence of amino acids (primary structure) determines how the protein folds into its three-dimensional structure. This folding is crucial for the protein's function. Protein structure is often described in four levels:
1. Primary Structure: The Amino Acid Sequence
The primary structure is simply the linear sequence of amino acids in the polypeptide chain. This sequence is dictated by the genetic code, with the DNA sequence determining the order of amino acids during protein synthesis. Even a small change in this sequence, such as a single amino acid substitution, can drastically alter the protein's structure and function, as seen in sickle cell anemia.
2. Secondary Structure: Local Folding Patterns
The primary structure then folds into local patterns stabilized by hydrogen bonds between the amino and carboxyl groups of the peptide backbone. The most common secondary structures are:
- Alpha-helices: A right-handed coiled structure stabilized by hydrogen bonds between every fourth amino acid.
- Beta-sheets: Extended polypeptide chains arranged side-by-side, forming a sheet-like structure stabilized by hydrogen bonds between adjacent strands. These can be parallel or antiparallel, depending on the orientation of the strands.
3. Tertiary Structure: The 3D Arrangement
The tertiary structure refers to the overall three-dimensional arrangement of a polypeptide chain, encompassing all secondary structure elements. It is stabilized by a variety of interactions, including:
- Hydrophobic interactions: Nonpolar amino acid side chains cluster together in the protein's interior, avoiding contact with water.
- Hydrogen bonds: Interactions between polar side chains and/or the peptide backbone.
- Ionic bonds (salt bridges): Electrostatic interactions between oppositely charged side chains.
- Disulfide bonds: Covalent bonds between cysteine residues, creating strong cross-links within the protein.
The tertiary structure is crucial for the protein's function. The specific arrangement of amino acids creates active sites in enzymes, binding sites for other molecules, and structural features necessary for the protein's role in the cell.
4. Quaternary Structure: Multi-subunit Proteins
Some proteins consist of multiple polypeptide chains (subunits), each with its own tertiary structure. The arrangement of these subunits constitutes the quaternary structure. Examples include hemoglobin, which has four subunits, and many enzymes that require multiple subunits for activity. The interactions between subunits are similar to those stabilizing tertiary structure.
Modifications After Synthesis: Post-translational Modifications
Once synthesized, proteins can undergo various post-translational modifications (PTMs) that alter their structure and function. These modifications can include:
- Glycosylation: The addition of sugar molecules.
- Phosphorylation: The addition of phosphate groups.
- Ubiquitination: The attachment of ubiquitin molecules, often targeting proteins for degradation.
- Acetylation: The addition of acetyl groups.
These modifications are crucial for regulating protein activity, localization, and stability.
The Importance of Amino Acid Sequence: Dictating Protein Function
The precise sequence of amino acids is paramount. A change in even one amino acid can significantly impact the protein's structure and function. This can lead to:
- Loss of function: The protein might be unable to perform its intended role.
- Gain of function: The protein might acquire a new, potentially harmful, function.
- Disease: Genetic mutations that alter amino acid sequences are often the cause of various diseases, including sickle cell anemia, cystic fibrosis, and many others.
Understanding the relationship between amino acid sequence, protein structure, and function is essential for comprehending numerous biological processes and for developing effective treatments for protein-related diseases.
Conclusion: Amino Acids – The Foundation of Life's Complexity
In essence, proteins are intricate macromolecules built from a relatively small set of monomers: the 20 standard amino acids. These amino acids, with their diverse properties, combine in countless sequences, creating a vast repertoire of proteins with diverse structures and functions. The precise amino acid sequence dictates the protein's three-dimensional structure, which, in turn, determines its biological activity. Understanding the relationship between amino acids, protein structure, and function is key to understanding the intricacies of life itself and developing strategies for addressing various diseases related to protein dysfunction. Further research continues to uncover the complexities of protein folding, interaction, and modifications, promising advances in medicine, biotechnology, and many other fields.
Latest Posts
Latest Posts
-
3 1 2 In Improper Fraction
May 13, 2025
-
How To Find Charge Of Polyatomic Ions
May 13, 2025
-
Find The Limit If It Exists
May 13, 2025
-
Expression That Represents The Area Of A Rectangle
May 13, 2025
-
Atomic Radius Increases From Left To Right
May 13, 2025
Related Post
Thank you for visiting our website which covers about What Is The Monomer Used To Make Protein . We hope the information provided has been useful to you. Feel free to contact us if you have any questions or need further assistance. See you next time and don't miss to bookmark.