What Is The Chemical Equation For Photosynthesis And Cellular Respiration
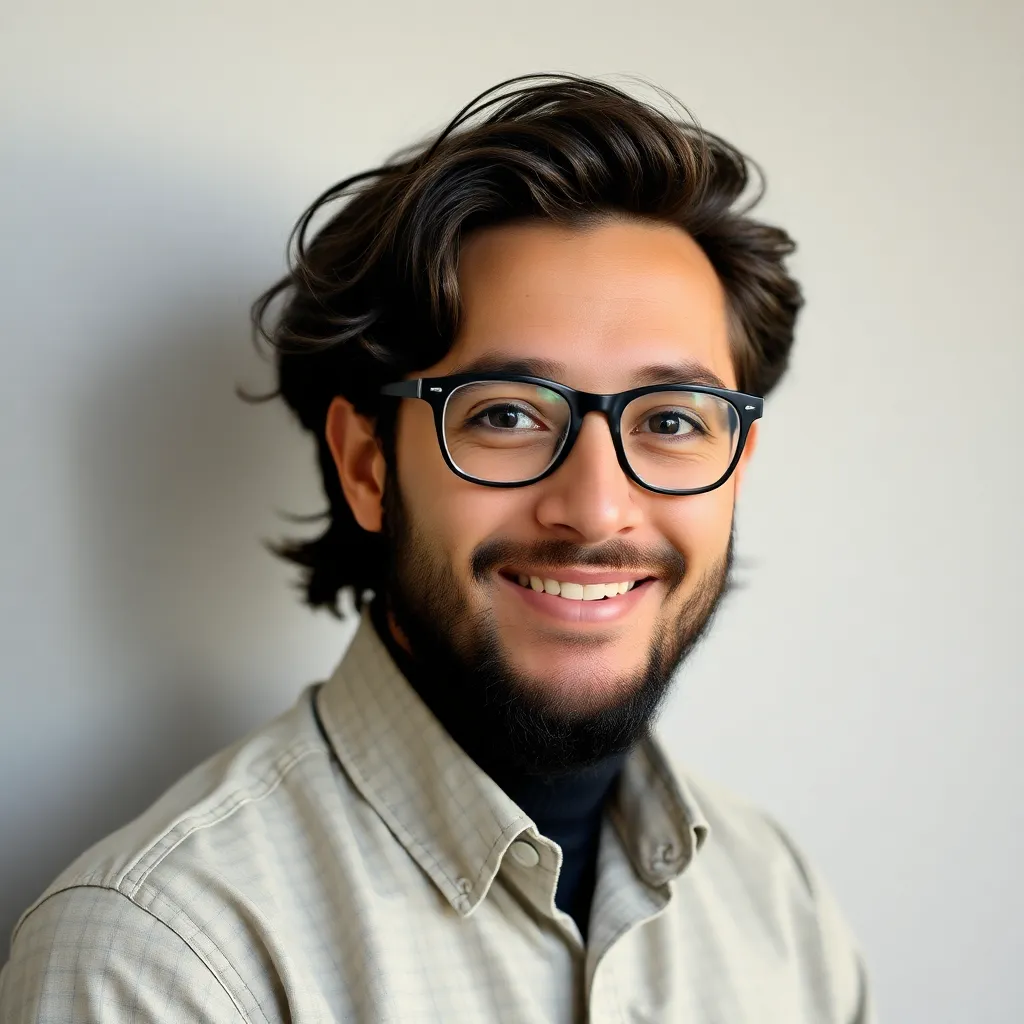
listenit
May 12, 2025 · 6 min read
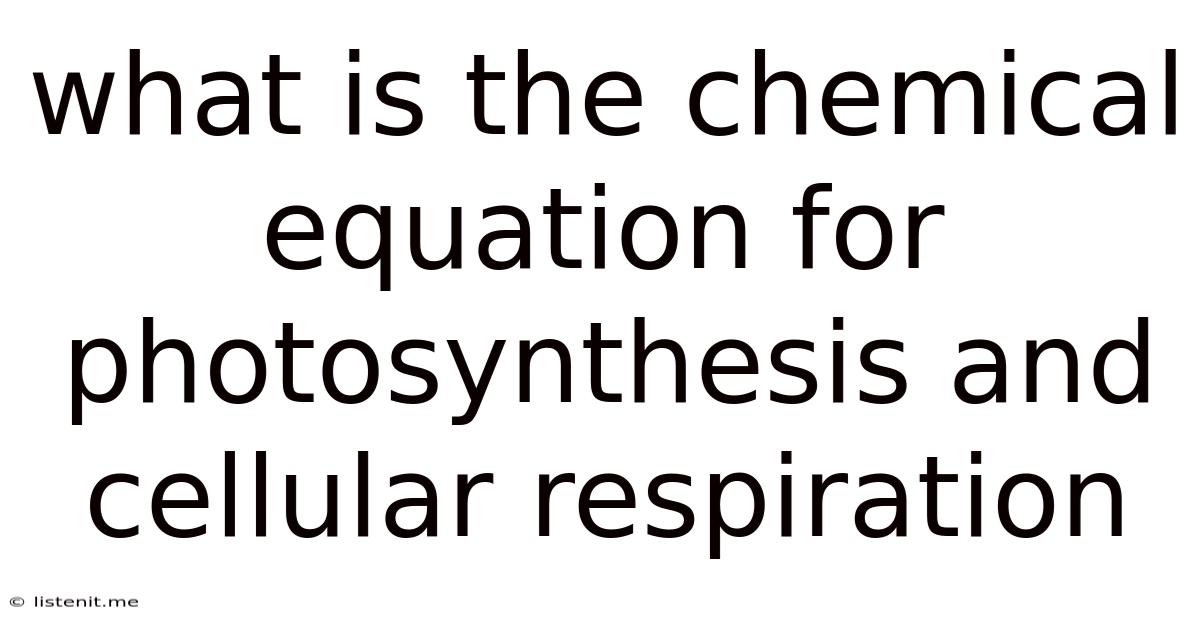
Table of Contents
What is the Chemical Equation for Photosynthesis and Cellular Respiration?
Understanding the chemical equations for photosynthesis and cellular respiration is fundamental to grasping the intricate dance of energy transfer within the biological world. These two processes are essentially opposites, with photosynthesis capturing solar energy to create fuel, and cellular respiration breaking down that fuel to release energy for cellular work. Let's delve deep into each process, exploring their chemical equations, intricacies, and their crucial roles in maintaining life on Earth.
Photosynthesis: Capturing Sunlight's Energy
Photosynthesis is the remarkable process by which green plants, algae, and some bacteria convert light energy into chemical energy in the form of glucose. This process is the cornerstone of most food chains, providing the energy base for almost all ecosystems. The overall chemical equation for photosynthesis is often simplified as:
6CO₂ + 6H₂O + Light Energy → C₆H₁₂O₆ + 6O₂
This equation tells us that six molecules of carbon dioxide (CO₂) and six molecules of water (H₂O), in the presence of light energy, react to produce one molecule of glucose (C₆H₁₂O₆) and six molecules of oxygen (O₂). However, this simplified equation masks the incredible complexity of the process, which unfolds in two main stages: the light-dependent reactions and the light-independent reactions (also known as the Calvin cycle).
The Light-Dependent Reactions: Harvesting Light Energy
The light-dependent reactions occur within the thylakoid membranes of chloroplasts. Chlorophyll and other pigments absorb light energy, exciting electrons to a higher energy level. This energy is then used to:
- Split water molecules (photolysis): This process releases electrons, protons (H⁺), and oxygen (O₂). The oxygen is released as a byproduct.
- Generate ATP (adenosine triphosphate): ATP is the cell's primary energy currency. The energy from excited electrons is used to generate a proton gradient across the thylakoid membrane, driving ATP synthesis through chemiosmosis.
- Produce NADPH: NADPH is a reducing agent, carrying high-energy electrons to the next stage of photosynthesis.
These reactions are complex, involving multiple protein complexes and electron carriers, but the net result is the conversion of light energy into the chemical energy stored in ATP and NADPH.
The Light-Independent Reactions (Calvin Cycle): Building Glucose
The light-independent reactions, or Calvin cycle, take place in the stroma of the chloroplasts. Here, the ATP and NADPH produced during the light-dependent reactions are used to fix carbon dioxide and synthesize glucose. The cycle involves a series of enzyme-catalyzed reactions, with the key steps including:
- Carbon fixation: CO₂ is incorporated into a five-carbon molecule (RuBP) by the enzyme RuBisCO, forming a six-carbon intermediate that quickly splits into two three-carbon molecules (3-PGA).
- Reduction: ATP and NADPH are used to reduce 3-PGA to glyceraldehyde-3-phosphate (G3P), a three-carbon sugar.
- Regeneration of RuBP: Some G3P molecules are used to regenerate RuBP, ensuring the cycle continues.
- Glucose synthesis: Other G3P molecules are used to synthesize glucose and other sugars.
The Calvin cycle is a cyclical process, constantly regenerating the molecules needed to fix more CO₂. The net result is the conversion of inorganic carbon (CO₂) into the organic carbon of glucose, storing the energy captured from sunlight.
Factors Affecting Photosynthesis
Several factors can influence the rate of photosynthesis, including:
- Light intensity: Photosynthesis increases with light intensity up to a saturation point, beyond which further increases have no effect.
- Carbon dioxide concentration: Higher CO₂ levels generally increase photosynthetic rates.
- Temperature: Photosynthesis is optimal within a specific temperature range, with excessively high or low temperatures inhibiting the process.
- Water availability: Water is a reactant in photosynthesis, so its availability is crucial.
Understanding these factors is essential for optimizing plant growth and crop yields. Agricultural practices often focus on manipulating these factors to maximize photosynthetic efficiency.
Cellular Respiration: Releasing Energy from Glucose
Cellular respiration is the process by which cells break down glucose to release energy stored within its chemical bonds. This energy is then used to power various cellular activities, including growth, movement, and maintaining homeostasis. The overall chemical equation for cellular respiration is:
C₆H₁₂O₆ + 6O₂ → 6CO₂ + 6H₂O + Energy (ATP)
This equation shows that one molecule of glucose (C₆H₁₂O₆) reacts with six molecules of oxygen (O₂) to produce six molecules of carbon dioxide (CO₂), six molecules of water (H₂O), and a significant amount of energy in the form of ATP. Like photosynthesis, cellular respiration is a complex multi-step process, divided into four main stages: glycolysis, pyruvate oxidation, the Krebs cycle, and oxidative phosphorylation.
Glycolysis: Breaking Down Glucose
Glycolysis occurs in the cytoplasm and doesn't require oxygen. It involves the breakdown of glucose into two molecules of pyruvate. This process generates a small amount of ATP and NADH.
Pyruvate Oxidation: Preparing for the Krebs Cycle
Pyruvate, produced during glycolysis, is transported into the mitochondria, where it undergoes oxidation, converting into acetyl-CoA. This step also generates NADH and releases carbon dioxide.
The Krebs Cycle (Citric Acid Cycle): Extracting Energy
The Krebs cycle takes place in the mitochondrial matrix. Acetyl-CoA enters the cycle, undergoing a series of reactions that release carbon dioxide and generate ATP, NADH, and FADH₂ (another electron carrier).
Oxidative Phosphorylation: The Electron Transport Chain and Chemiosmosis
Oxidative phosphorylation is the final and most energy-yielding stage of cellular respiration. It occurs in the inner mitochondrial membrane. Electrons from NADH and FADH₂ are passed along the electron transport chain, a series of protein complexes. This electron flow drives the pumping of protons (H⁺) across the inner mitochondrial membrane, creating a proton gradient. This gradient drives ATP synthesis through chemiosmosis, generating a large amount of ATP. Oxygen acts as the final electron acceptor, forming water.
Types of Cellular Respiration
While the above describes aerobic cellular respiration (requiring oxygen), other forms exist:
- Anaerobic Respiration: Some organisms can generate ATP without oxygen through anaerobic respiration. This often involves fermentation pathways, such as lactic acid fermentation or alcoholic fermentation, which produce less ATP than aerobic respiration.
Factors Affecting Cellular Respiration
Various factors influence the rate of cellular respiration, including:
- Oxygen availability: Aerobic respiration requires oxygen; its absence dramatically reduces ATP production.
- Glucose availability: Glucose is the primary fuel source; its concentration affects the rate of respiration.
- Temperature: Enzyme activity is temperature-dependent, influencing the rate of each step.
- pH: Changes in pH can affect enzyme activity and the electron transport chain.
Photosynthesis and Cellular Respiration: A Symbiotic Relationship
Photosynthesis and cellular respiration are interconnected processes that form a crucial cycle in the biosphere. Photosynthesis captures solar energy and converts it into the chemical energy stored in glucose, releasing oxygen as a byproduct. Cellular respiration then uses this glucose to generate ATP, the energy currency of cells, releasing carbon dioxide and water. The carbon dioxide released during respiration is then used by plants during photosynthesis, and the oxygen produced by photosynthesis is used by organisms for respiration. This continuous cycle sustains life on Earth, balancing energy flow and the exchange of essential gases.
Conclusion
The chemical equations for photosynthesis and cellular respiration represent the core processes driving energy flow within ecosystems. While simplified equations provide a basic understanding, the actual processes are incredibly complex, involving intricate molecular mechanisms and a delicate interplay of factors. A thorough understanding of these processes is critical for addressing various challenges facing our planet, from developing sustainable agriculture practices to combating climate change. By exploring the intricacies of photosynthesis and cellular respiration, we gain a deeper appreciation for the fundamental processes that sustain life itself.
Latest Posts
Latest Posts
-
Write An Equation In Standard Form For The Circle
May 12, 2025
-
Abiotic And Biotic Factors In The Desert
May 12, 2025
-
Write The Complete Ground State Electron Configuration Of Chlorine
May 12, 2025
-
Least Common Multiple Of 32 And 48
May 12, 2025
-
The Thing That The Scientist Changes In An Experiment
May 12, 2025
Related Post
Thank you for visiting our website which covers about What Is The Chemical Equation For Photosynthesis And Cellular Respiration . We hope the information provided has been useful to you. Feel free to contact us if you have any questions or need further assistance. See you next time and don't miss to bookmark.