What Holds Strands Of Dna Together
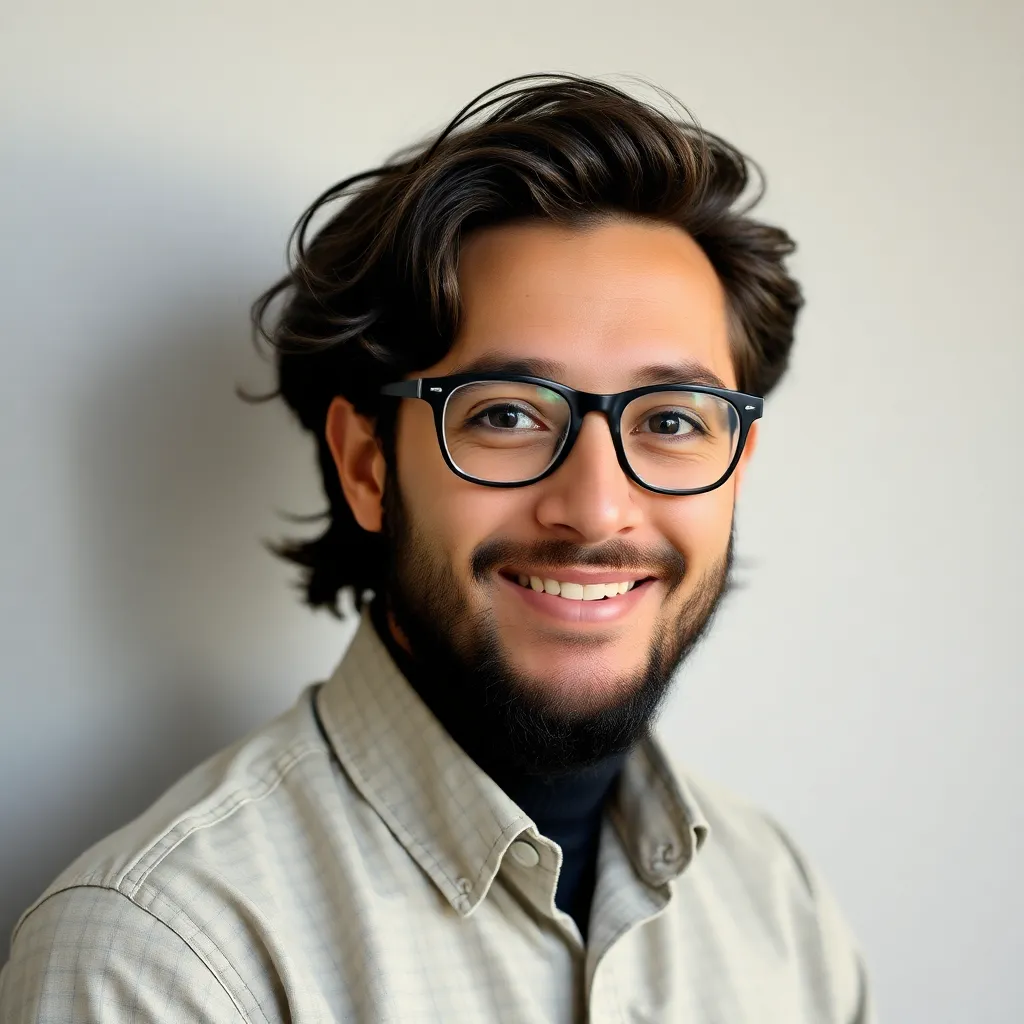
listenit
May 10, 2025 · 6 min read
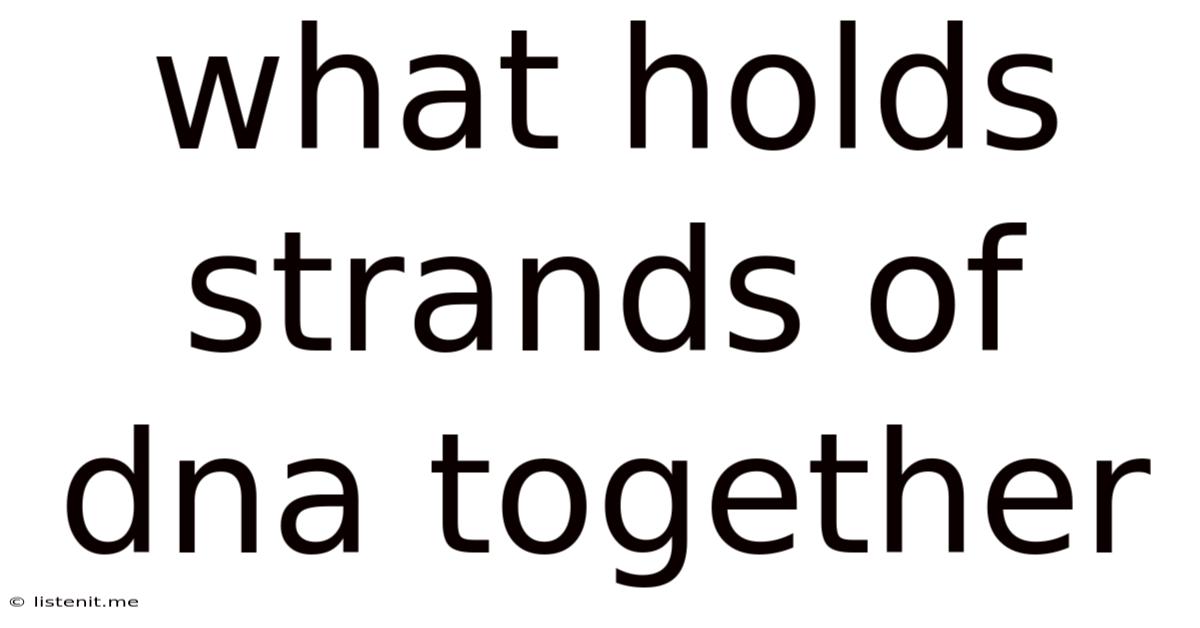
Table of Contents
What Holds Strands of DNA Together? The Intricate Dance of Hydrogen Bonds and Base Stacking
Deoxyribonucleic acid, or DNA, the very blueprint of life, is a remarkably elegant molecule. Its ability to store, replicate, and transmit genetic information relies heavily on the precise way its two strands are held together. This isn't a simple matter of sticking two pieces together; it's a sophisticated interplay of forces, primarily hydrogen bonds and base stacking interactions, that create the iconic double helix structure and ensure its stability. Understanding these forces is key to understanding life itself.
The Double Helix: A Masterpiece of Molecular Architecture
Before diving into the specifics of the bonds, let's briefly revisit the structure of DNA. DNA is a double helix, resembling a twisted ladder. The sides of this ladder are formed by sugar-phosphate backbones, while the "rungs" are formed by pairs of nitrogenous bases. These bases are the crucial players in the bonding process.
There are four types of nitrogenous bases in DNA: adenine (A), guanine (G), cytosine (C), and thymine (T). Crucially, these bases always pair up in a specific manner: adenine pairs with thymine (A-T), and guanine pairs with cytosine (G-C). This specific pairing is the foundation of DNA's stability and its ability to replicate accurately.
Hydrogen Bonds: The Glue that Holds the Base Pairs Together
The primary force holding the two DNA strands together is the hydrogen bond. These are relatively weak bonds compared to covalent bonds, but their collective strength is significant. Each base pair is held together by a specific number of hydrogen bonds:
-
Adenine (A) and Thymine (T): These bases form two hydrogen bonds. A hydrogen atom on the amino group of adenine is attracted to an oxygen atom on thymine, and another hydrogen atom on the amino group of adenine forms a bond with a nitrogen atom in thymine.
-
Guanine (G) and Cytosine (C): These bases form three hydrogen bonds. One hydrogen bond forms between a hydrogen atom on the amino group of guanine and an oxygen atom on cytosine. Two more hydrogen bonds occur between a hydrogen atom on guanine's carbonyl group and two different nitrogen atoms on cytosine.
The number of hydrogen bonds influences the stability of the base pairs. G-C pairs, with three hydrogen bonds, are generally stronger and more resistant to separation than A-T pairs, which have only two. This difference in strength has implications for DNA's melting temperature – the temperature at which the two strands separate – and its overall stability.
The Specificity of Hydrogen Bonding: Ensuring Accurate Replication
The precise arrangement of hydrogen bond donors and acceptors in each base ensures the specificity of base pairing. Adenine can only form hydrogen bonds with thymine, and guanine can only form hydrogen bonds with cytosine. This specificity is absolutely critical for accurate DNA replication. When the DNA molecule replicates, the two strands separate, and each strand serves as a template for the synthesis of a new complementary strand. The hydrogen bonding ensures that the correct bases are added to the new strand, maintaining the genetic information faithfully. Any mismatch in base pairing would lead to mutations and potentially harmful consequences.
Base Stacking: A Subtle but Crucial Force
While hydrogen bonds are the primary force holding the base pairs together, another crucial interaction contributes significantly to the overall stability of the DNA double helix: base stacking. This refers to the hydrophobic interactions between the stacked bases within the DNA helix.
The nitrogenous bases are relatively hydrophobic (water-repelling) molecules. They tend to cluster together in the interior of the DNA helix, away from the surrounding aqueous environment. This hydrophobic effect contributes significantly to the stability of the double helix. The bases are planar and stacked one on top of another, with their hydrophobic surfaces interacting favorably. This interaction is driven by van der Waals forces, which are weak but become substantial due to the cumulative effect of multiple stacked bases.
Optimizing Base Stacking for Stability
The specific geometry of the double helix is optimized to maximize base stacking interactions. The twisting of the helix allows for optimal overlap between the stacked bases, further stabilizing the structure. This stacking is not static; the bases can slightly shift and adjust their positions, contributing to the flexibility of the DNA molecule, but overall it provides a considerable contribution to the helix’s structural integrity.
Other Factors Affecting DNA Stability
While hydrogen bonds and base stacking are the dominant forces, other factors also influence the stability of the DNA double helix:
-
Electrostatic interactions: The negatively charged phosphate groups in the sugar-phosphate backbone repel each other. This repulsion tends to destabilize the double helix. However, this repulsion is counteracted to some extent by the positive charges of metal ions (like Mg²⁺) that neutralize some of the negative charge.
-
Solvent effects: The surrounding aqueous environment plays a role in DNA stability. Changes in the ionic strength or pH of the solution can significantly affect the strength of the interactions within the DNA molecule.
-
DNA Supercoiling: The DNA molecule can be further compacted by supercoiling, which introduces additional twists into the double helix. Supercoiling can affect the accessibility of DNA to enzymes and other proteins that interact with it.
The Dynamic Nature of DNA
It's important to remember that the DNA double helix is not a rigid, static structure. It’s a highly dynamic molecule. The bases can slightly shift their positions, the double helix can bend and flex, and the strands can even transiently separate in certain regions. This flexibility is crucial for the many processes that involve DNA, such as transcription (the synthesis of RNA from DNA) and replication. Enzymes that interact with DNA, such as DNA polymerases and helicases, exploit these dynamic properties to carry out their functions.
Implications for DNA Research and Biotechnology
Understanding the forces that hold DNA strands together has profound implications for various fields of research and technology. This knowledge is critical for:
-
Developing new gene editing technologies: Techniques like CRISPR-Cas9 rely on the ability to precisely cut and modify DNA. Understanding the strength and specificity of base pairing and stacking is vital for optimizing these technologies.
-
Drug development: Many drugs target DNA, either by inhibiting replication or by interacting with specific DNA sequences. Understanding the interactions that stabilize the DNA double helix can lead to the development of more effective drugs.
-
Forensic science: DNA fingerprinting relies on the ability to separate and analyze DNA fragments. Understanding DNA stability is essential for optimizing DNA extraction, amplification, and analysis procedures.
-
Nanotechnology: DNA's unique properties, including its self-assembly capabilities, are being explored in nanotechnology for developing novel materials and devices. Understanding the interactions holding the DNA double helix together is crucial for designing and controlling the self-assembly of DNA nanostructures.
Conclusion: A Delicate Balance of Forces
The stability of the DNA double helix is a delicate balance between several forces. The hydrogen bonds between base pairs provide the primary interaction, holding the two strands together. Base stacking interactions, driven by hydrophobic effects and van der Waals forces, contribute significantly to the stability. Other factors, such as electrostatic interactions and solvent effects, also play a role. This intricate interplay of forces allows DNA to store and transmit genetic information with remarkable fidelity, underpinning the very basis of life. Further research into the intricacies of these interactions will continue to unlock deeper insights into the workings of life and pave the way for groundbreaking technological advancements.
Latest Posts
Latest Posts
-
Ax By C Solve For A
May 11, 2025
-
What Percent Of 300 Is 90
May 11, 2025
-
What Do Plant Animal And Bacterial Cells Have In Common
May 11, 2025
-
Moment Generating Function Of Chi Square Distribution
May 11, 2025
-
What Are Valence Electrons Why Are They Important
May 11, 2025
Related Post
Thank you for visiting our website which covers about What Holds Strands Of Dna Together . We hope the information provided has been useful to you. Feel free to contact us if you have any questions or need further assistance. See you next time and don't miss to bookmark.