What Happens When Two Protons Get Closer Together
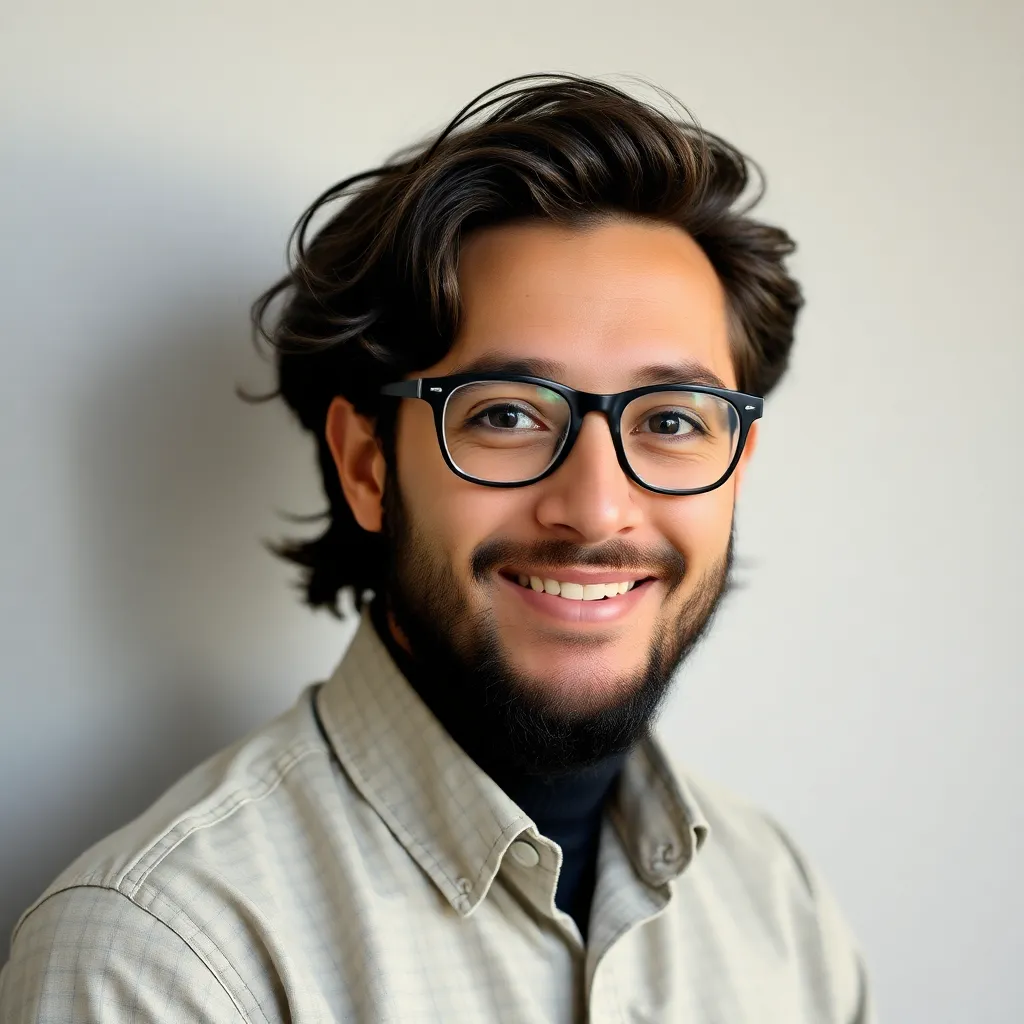
listenit
May 10, 2025 · 6 min read
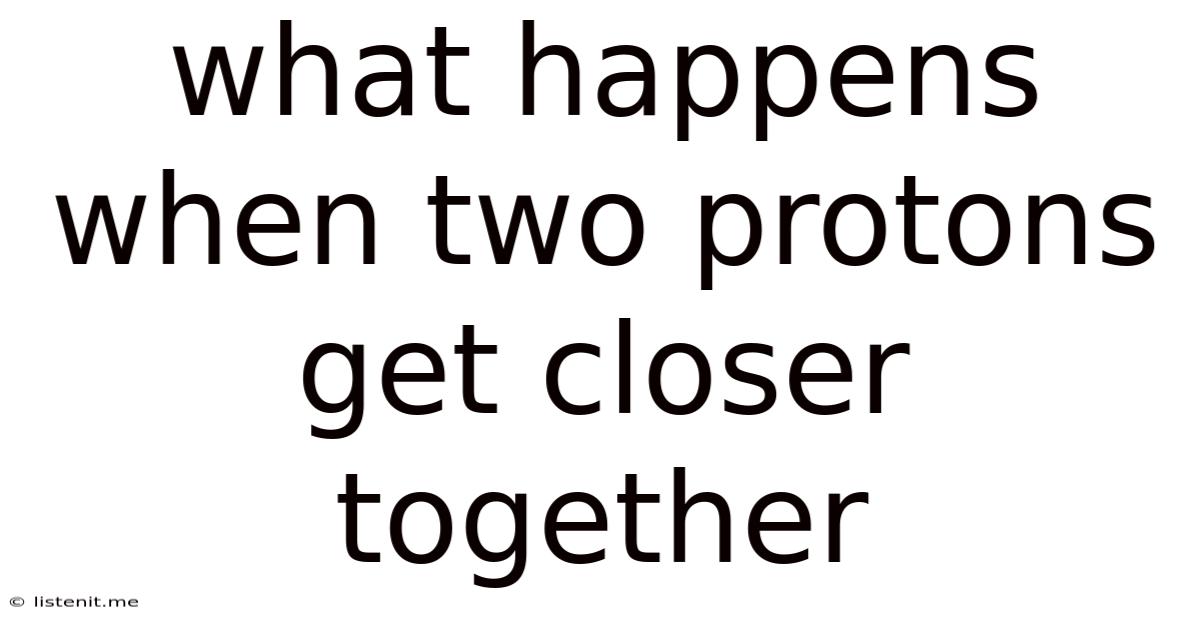
Table of Contents
What Happens When Two Protons Get Closer Together? A Deep Dive into the Strong Nuclear Force
The seemingly simple question, "What happens when two protons get closer together?" opens a door to a fascinating world of fundamental forces, quantum mechanics, and the very fabric of matter. It's a journey that takes us from the macroscopic world we experience daily to the subatomic realm governed by laws far removed from our everyday intuition. This exploration will delve into the intricacies of the strong nuclear force, the electromagnetic force, and the quantum tunneling effect, unraveling the complex interplay that determines the fate of approaching protons.
The Electromagnetic Repulsion: An Initial Hurdle
Before we explore the attractive forces, it's crucial to acknowledge the significant electromagnetic repulsion that immediately comes into play when two protons approach each other. Protons, as positively charged particles, experience a powerful repulsive force governed by Coulomb's Law. This law dictates that the force is directly proportional to the product of their charges and inversely proportional to the square of the distance between them. The closer the protons get, the stronger this repulsive force becomes, acting as a significant barrier to their union. This repulsion is a dominant force at distances greater than a few femtometers (1 femtometer = 10<sup>-15</sup> meters).
Overcoming the Coulomb Barrier: The Role of Kinetic Energy
To get closer, the protons need sufficient kinetic energy to overcome this electromagnetic repulsion. This kinetic energy can be provided through various processes, such as in nuclear reactions within stars or particle accelerators. The minimum kinetic energy required to bring the protons close enough for the strong nuclear force to take over is often referred to as the Coulomb barrier. The height of this barrier depends on the protons' initial energy and the distance they need to traverse. This barrier is a crucial factor in understanding nuclear fusion processes, as it explains why high temperatures and pressures are necessary to initiate fusion reactions.
The Strong Nuclear Force: A Short-Range Attraction
Once the protons overcome the initial electromagnetic repulsion and reach a distance of approximately 1 femtometer, a new force comes into play: the strong nuclear force. This force is fundamentally different from the electromagnetic force; it's much stronger but acts only over extremely short distances. The strong force is responsible for binding protons and neutrons together within the atomic nucleus, overcoming the electromagnetic repulsion between the positively charged protons.
Gluons and Quarks: The Mediators of the Strong Force
The strong force is mediated by particles called gluons, which are exchanged between the quarks that constitute protons and neutrons. Protons and neutrons are not fundamental particles; they are composed of three quarks each (two up quarks and one down quark for a proton, and two down quarks and one up quark for a neutron). Gluons carry the strong force, much like photons carry the electromagnetic force. The strong interaction between quarks and gluons is described by a complex theory called Quantum Chromodynamics (QCD).
Asymptotic Freedom and Confinement: Two Sides of the Strong Force
QCD exhibits two remarkable properties: asymptotic freedom and confinement. Asymptotic freedom means that at very short distances, the strong force between quarks becomes weak. This allows quarks to behave almost as free particles within the proton. Confinement, on the other hand, means that quarks cannot be isolated; they are always bound together in composite particles like protons and neutrons. This is why we never observe free quarks in nature. The strong force's behavior at these incredibly small distances is a complex area of ongoing research in particle physics.
Quantum Tunneling: A Probabilistic Leap
Even with sufficient kinetic energy to overcome the Coulomb barrier, the protons might still not fuse. This is because of the probabilistic nature of quantum mechanics. A phenomenon called quantum tunneling allows the protons to have a non-zero probability of penetrating the Coulomb barrier even if they don't possess enough energy to classically overcome it. This is a consequence of the wave-particle duality of matter, where particles can behave as waves and exhibit wave-like properties like tunneling through potential barriers. The probability of tunneling depends on the height and width of the barrier and the energy of the protons.
The Role of Quantum Tunneling in Nuclear Fusion
Quantum tunneling is crucial for nuclear fusion reactions, particularly in stars. In the core of stars, where temperatures and pressures are extremely high, the kinetic energy of protons is significantly increased, but it's still not enough to overcome the Coulomb barrier for all the protons. However, quantum tunneling enables a significant portion of protons to penetrate the barrier, leading to fusion reactions. Without quantum tunneling, stellar nucleosynthesis – the process that creates heavier elements from lighter ones – would be far less efficient.
What Happens After the Protons Get Close Enough?
If the protons overcome the Coulomb barrier and get close enough, the strong nuclear force becomes dominant. This leads to several possibilities:
Fusion: Forming Deuterium
In one scenario, the two protons can fuse together. However, this fusion is not a simple merging. One proton undergoes beta-plus decay, converting a proton into a neutron, releasing a positron (anti-electron) and an electron neutrino. The resulting nucleus is a deuteron, composed of one proton and one neutron – an isotope of hydrogen called deuterium. This fusion process releases a significant amount of energy, mainly in the form of kinetic energy of the emitted particles.
Elastic Scattering: Bouncing Off
In another scenario, the protons may simply scatter elastically, meaning they interact but don't fuse. They exchange energy and momentum but remain as individual protons, essentially bouncing off each other. The probability of elastic scattering versus fusion depends on several factors, including the protons' kinetic energy and their relative orientation.
Other Reactions: A Complex Landscape
The interaction of two protons at close range can also lead to other, more complex reactions, especially at higher energies. These reactions can involve the production of other particles or resonances, further highlighting the complex interplay between the electromagnetic and strong nuclear forces.
Conclusion: A Dance of Forces
The interaction between two approaching protons is a fascinating example of the interplay between fundamental forces in nature. The electromagnetic repulsion acts as an initial hurdle, but the strong nuclear force, once it comes into effect, can overcome this repulsion, leading to fusion or other interactions. Quantum tunneling plays a crucial role in enabling fusion even when the kinetic energy isn't sufficient to classically overcome the Coulomb barrier. The outcome of this interaction depends on a complex interplay of factors governed by the laws of quantum mechanics and the nature of the strong nuclear force. Understanding this intricate dance of forces is fundamental to our understanding of nuclear physics, astrophysics, and the evolution of the universe itself. The journey from initial repulsion to potential fusion is a testament to the elegance and complexity of the physical world at its most fundamental level.
Latest Posts
Latest Posts
-
32 Out Of 50 Is What Percent
May 10, 2025
-
What Is 9 20 In Decimal Form
May 10, 2025
-
How Does Hydrogen Bonding Affect Boiling Point
May 10, 2025
-
Centers Of Low Pressure Are Called
May 10, 2025
-
Where Is The Setting Of Beowulf
May 10, 2025
Related Post
Thank you for visiting our website which covers about What Happens When Two Protons Get Closer Together . We hope the information provided has been useful to you. Feel free to contact us if you have any questions or need further assistance. See you next time and don't miss to bookmark.