What Happens To Potential Energy As Kinetic Energy Increases
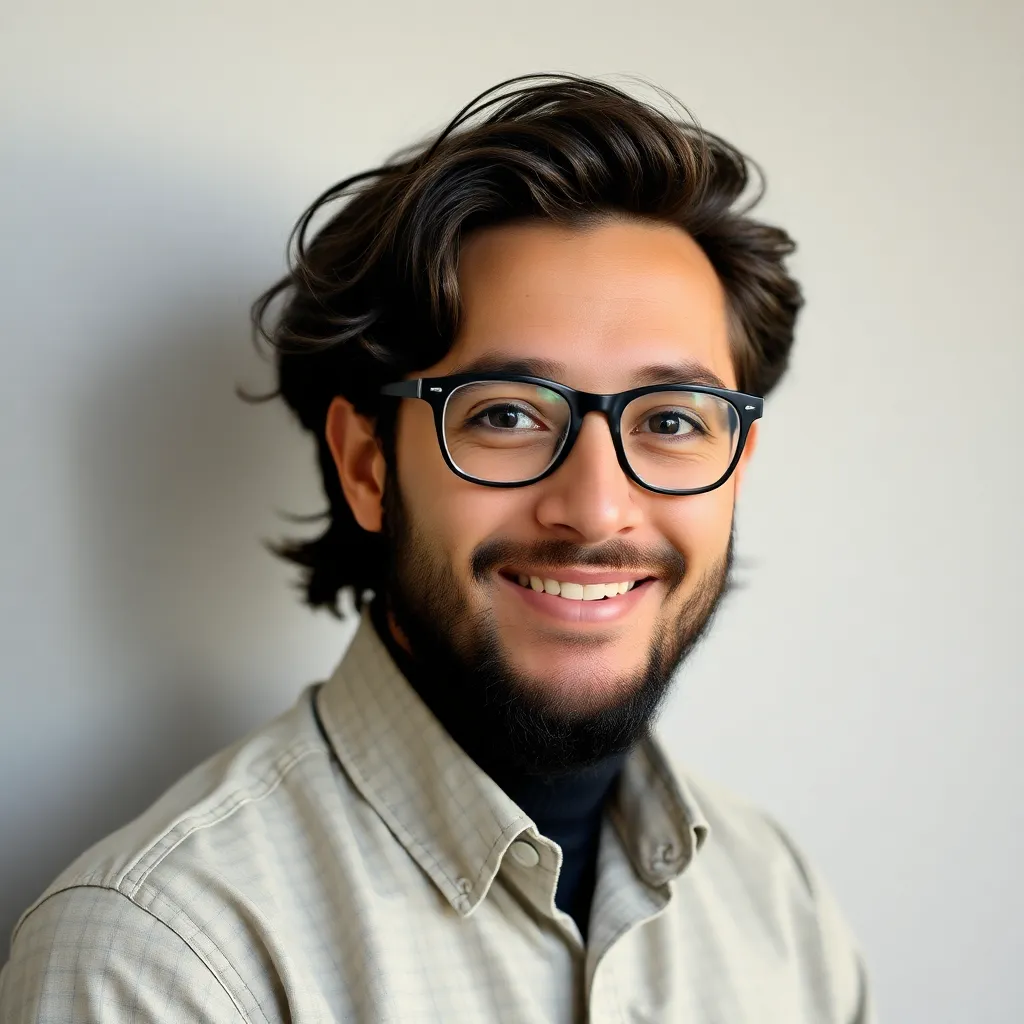
listenit
May 12, 2025 · 6 min read
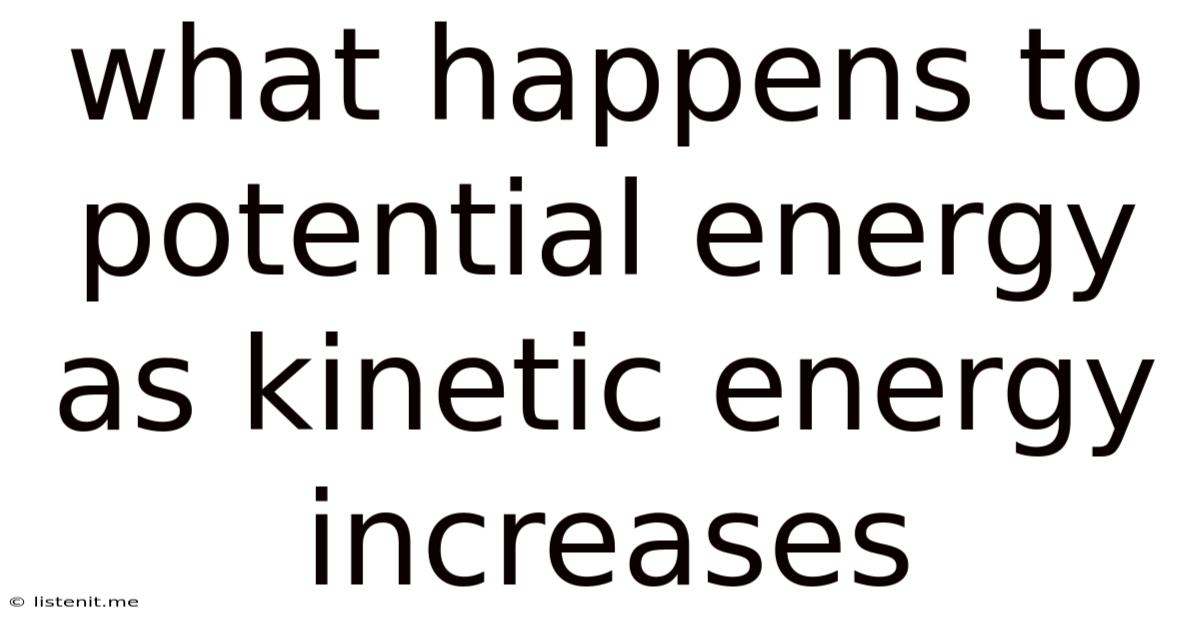
Table of Contents
What Happens to Potential Energy as Kinetic Energy Increases?
The relationship between potential energy and kinetic energy is fundamental to understanding how energy transforms within a system. This article will explore this dynamic relationship in detail, examining various scenarios and illustrating the principles with real-world examples. We'll delve into the conservation of energy, exploring exceptions and nuanced situations to provide a comprehensive understanding of this crucial concept in physics.
The Interplay of Potential and Kinetic Energy
The core principle governing the interaction of potential and kinetic energy is the law of conservation of energy. This law states that energy cannot be created or destroyed, only transformed from one form to another. In a closed system, the total mechanical energy (the sum of potential and kinetic energy) remains constant.
Potential energy (PE) is stored energy that has the potential to be converted into other forms of energy. This energy is often associated with an object's position or configuration within a force field (like gravity or an elastic field). Examples include:
- Gravitational potential energy: An object held at a height above the ground possesses gravitational potential energy. The higher the object, the greater its potential energy.
- Elastic potential energy: A stretched spring or a compressed rubber band stores elastic potential energy.
- Chemical potential energy: The energy stored in the bonds of molecules, like in gasoline or food.
Kinetic energy (KE) is the energy of motion. Any object in motion possesses kinetic energy. The faster the object moves, and the greater its mass, the greater its kinetic energy. The formula for kinetic energy is:
KE = 1/2 * mv²
where 'm' is the mass and 'v' is the velocity.
Transformations: A Closer Look
As potential energy decreases, kinetic energy increases, and vice-versa, maintaining a constant total mechanical energy within an ideal system (neglecting energy loss due to friction or other dissipative forces). Let's examine some classic examples:
1. Falling Object
Consider a ball held at a height above the ground. Initially, it possesses maximum gravitational potential energy and zero kinetic energy (it's not moving). As the ball is released, it falls towards the earth. Its potential energy gradually converts into kinetic energy. As it falls, its speed increases, leading to a rise in kinetic energy. Just before impact, the ball has minimal potential energy (near zero at ground level) and maximum kinetic energy.
Factors Affecting the Transformation:
- Height: A greater initial height results in a larger conversion of potential to kinetic energy, leading to a higher final velocity.
- Mass: A heavier ball will have a greater kinetic energy at any given velocity compared to a lighter ball.
- Air Resistance: Air resistance (a dissipative force) opposes the motion of the falling ball, converting some of its kinetic energy into heat. In reality, the total mechanical energy is not perfectly conserved due to this energy loss.
2. Roller Coaster
A roller coaster provides a more complex illustration of the potential and kinetic energy interplay. At the highest point of the track, the coaster has maximum potential energy and minimal kinetic energy (it's moving slowly). As it descends, potential energy converts to kinetic energy, increasing its speed. At the bottom of the hill, it has maximum kinetic energy and minimal potential energy. The coaster then ascends another hill, converting kinetic energy back into potential energy, slowing down as it climbs. This process of energy transformation continues throughout the ride.
Energy Losses in Roller Coasters:
Roller coasters inevitably experience energy loss due to friction between the wheels and the track, air resistance, and other dissipative forces. This loss manifests as heat, reducing the coaster's total mechanical energy over the course of the ride. To compensate, the initial ascent often utilizes a chain lift or other mechanical means to add potential energy to the system.
3. Pendulum Swing
A simple pendulum provides another elegant example. At its highest point, the pendulum bob possesses maximum potential energy and zero kinetic energy (it momentarily stops before changing direction). As it swings down, potential energy converts to kinetic energy, reaching its maximum kinetic energy at the bottom of its swing. As it swings upwards again, kinetic energy converts back into potential energy, slowing until it momentarily stops at the highest point on the other side, and the cycle repeats.
Ideal vs. Real Pendulum:
An ideal pendulum, without friction or air resistance, would continue swinging indefinitely, conserving its total mechanical energy. However, a real pendulum loses energy due to friction at the pivot point and air resistance, gradually reducing its amplitude of swing until it comes to rest.
4. Spring-Mass System
A mass attached to a spring exemplifies the transformation between elastic potential energy and kinetic energy. When the spring is stretched or compressed, it stores elastic potential energy. Upon release, the spring's potential energy is converted into the kinetic energy of the mass, causing it to oscillate back and forth. The mass moves fastest when the spring is at its equilibrium position (where the elastic potential energy is zero). At the maximum displacement, the kinetic energy is zero, and the elastic potential energy is maximum.
Damping in Spring-Mass Systems:
Like other real-world systems, the spring-mass system also experiences energy loss due to friction (internal friction within the spring and friction between the mass and its surroundings), and air resistance. This damping effect gradually reduces the amplitude of oscillation until the system comes to rest.
Beyond Simple Systems: Considering Complexities
The examples above illustrate the fundamental principle in relatively simple systems. However, in more complex situations, other forms of energy come into play, further complicating the relationship between potential and kinetic energy.
Non-Conservative Forces
Forces that dissipate energy (like friction and air resistance) are called non-conservative forces. These forces convert mechanical energy into other forms, usually heat, making the total mechanical energy of a system decrease over time.
Internal Energy
The internal energy of a system refers to the kinetic and potential energy of the molecules within the system. When non-conservative forces act, some mechanical energy is converted into internal energy, increasing the temperature of the system.
Other Forms of Energy
Many systems involve transformations between mechanical energy and other forms of energy, such as:
- Electrical energy: A hydroelectric dam converts gravitational potential energy into electrical energy.
- Chemical energy: Burning fuel converts chemical potential energy into thermal energy and kinetic energy.
- Nuclear energy: Nuclear reactions convert nuclear potential energy into other forms of energy.
Conclusion: A Dynamic Equilibrium
The relationship between potential and kinetic energy is a dynamic one, characterized by continuous transformation and, in real-world scenarios, energy dissipation. While the law of conservation of energy dictates that the total energy within a closed system remains constant, the distribution between potential and kinetic energy is constantly shifting. Understanding this interplay is crucial to comprehending a wide range of physical phenomena, from the simple motion of a falling object to the complex workings of machinery and natural processes. Acknowledging the impact of non-conservative forces and other energy forms provides a more realistic and complete understanding of energy transformations in the world around us. By considering these factors, we can develop a more nuanced understanding of the intricate dance between potential and kinetic energy.
Latest Posts
Latest Posts
-
Does Higher Ksp Mean More Soluble
May 12, 2025
-
How To Divide A Square Root By 2
May 12, 2025
-
Calculate The De Broglie Wavelength Of The Bullet
May 12, 2025
-
Finding Nth Term In Geometric Sequence
May 12, 2025
-
Write Each Polynomial In Standard Form
May 12, 2025
Related Post
Thank you for visiting our website which covers about What Happens To Potential Energy As Kinetic Energy Increases . We hope the information provided has been useful to you. Feel free to contact us if you have any questions or need further assistance. See you next time and don't miss to bookmark.