What Does Negative Gibbs Free Energy Mean
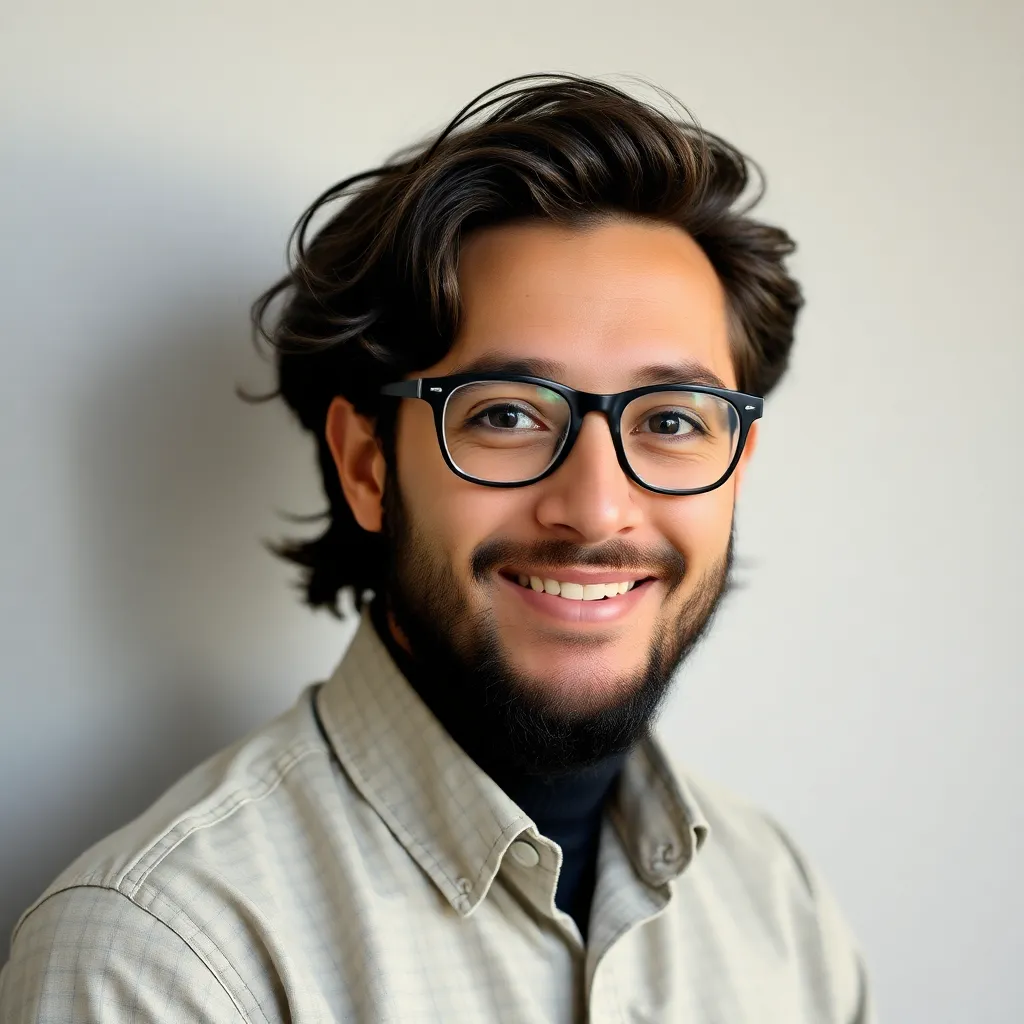
listenit
Apr 11, 2025 · 7 min read

Table of Contents
What Does Negative Gibbs Free Energy Mean? Understanding Spontaneity in Chemical Reactions
The concept of Gibbs Free Energy (ΔG) is fundamental to understanding the spontaneity of chemical and physical processes. A negative Gibbs Free Energy value signifies a reaction that will proceed spontaneously under constant temperature and pressure conditions. But what does this really mean, and why is it so crucial in chemistry, biochemistry, and other scientific fields? Let's delve deep into this important thermodynamic concept.
Understanding Gibbs Free Energy: A Deeper Dive
Gibbs Free Energy, named after the American mathematician Josiah Willard Gibbs, is a thermodynamic potential that measures the maximum reversible work that may be performed by a thermodynamic system at a constant temperature and pressure. It's a powerful tool because it combines enthalpy (ΔH), a measure of heat content, and entropy (ΔS), a measure of disorder, to predict the spontaneity of a reaction. The equation defining Gibbs Free Energy is:
ΔG = ΔH - TΔS
Where:
- ΔG is the change in Gibbs Free Energy (in Joules or Kilojoules)
- ΔH is the change in enthalpy (in Joules or Kilojoules)
- T is the absolute temperature (in Kelvin)
- ΔS is the change in entropy (in Joules per Kelvin)
The Significance of a Negative ΔG
A negative ΔG indicates that a reaction or process is spontaneous under the given conditions. This doesn't necessarily mean the reaction will be fast; spontaneity only refers to the thermodynamic favorability of the process. A spontaneous reaction can proceed without external input of energy. However, the rate at which it proceeds depends on the kinetics of the reaction (activation energy, reaction mechanisms, etc.), which is a separate concept.
Several scenarios can lead to a negative ΔG:
1. Exothermic Reactions with Increasing Entropy (ΔH < 0, ΔS > 0)
These reactions are the easiest to understand. They release heat (exothermic, ΔH < 0), and the products are more disordered than the reactants (positive entropy change, ΔS > 0). Both terms in the Gibbs Free Energy equation contribute to a negative ΔG, making the reaction highly spontaneous. A classic example is the combustion of fuels, where heat is released, and the products (gases) are much more disordered than the reactants (solid fuel and oxygen gas).
2. Exothermic Reactions with Decreasing Entropy (ΔH < 0, ΔS < 0)
In these cases, the reaction releases heat, but the products are more ordered than the reactants (negative entropy change). The spontaneity depends on the relative magnitudes of ΔH and TΔS. At low temperatures, the negative ΔH term dominates, leading to a negative ΔG and spontaneity. However, as temperature increases, the TΔS term (which is now positive) becomes more significant. At a sufficiently high temperature, TΔS could outweigh ΔH, resulting in a positive ΔG and non-spontaneity. The formation of some crystals from solution is an example; the process is exothermic but leads to a decrease in entropy.
3. Endothermic Reactions with Increasing Entropy (ΔH > 0, ΔS > 0)
These reactions absorb heat (endothermic, ΔH > 0) but have an increase in disorder (ΔS > 0). Spontaneity depends on the temperature. At low temperatures, the positive ΔH term dominates, resulting in a positive ΔG (non-spontaneous). However, at sufficiently high temperatures, the positive TΔS term can outweigh the positive ΔH, leading to a negative ΔG and spontaneity. The melting of ice is a prime example; it absorbs heat but increases the disorder of the system.
4. Endothermic Reactions with Decreasing Entropy (ΔH > 0, ΔS < 0)
These reactions are always non-spontaneous (positive ΔG) under all conditions. They absorb heat, and the products are more ordered than the reactants. No amount of temperature increase can make these reactions spontaneous because both terms in the Gibbs Free Energy equation contribute to a positive ΔG.
Implications of a Negative Gibbs Free Energy
The implications of a negative ΔG extend far beyond simple chemical reactions:
-
Predicting Reaction Direction: Knowing the sign of ΔG allows us to predict the direction a reaction will proceed to reach equilibrium. A negative ΔG indicates that the reaction will proceed spontaneously in the forward direction.
-
Equilibrium Constant: The Gibbs Free Energy is directly related to the equilibrium constant (K) of a reversible reaction through the equation:
ΔG° = -RTlnK
Where:
- ΔG° is the standard Gibbs Free Energy change
- R is the ideal gas constant
- T is the absolute temperature
- K is the equilibrium constant
A large negative ΔG° corresponds to a large equilibrium constant, indicating that the equilibrium lies far to the right (favoring product formation).
-
Biochemistry and Metabolism: Gibbs Free Energy is crucial in biochemistry, where it helps understand metabolic pathways. Many biochemical reactions are coupled—a spontaneous reaction (negative ΔG) is used to drive a non-spontaneous reaction (positive ΔG). The overall process remains spontaneous if the sum of the ΔG values is negative.
-
Material Science: The concept is essential in material science to predict the stability of materials and the feasibility of various processes like crystal growth, phase transformations, and alloy formation.
-
Environmental Science: Understanding spontaneity aids in analyzing environmental processes, predicting the fate of pollutants, and assessing the feasibility of remediation strategies.
Standard Gibbs Free Energy Change (ΔG°)
The standard Gibbs Free Energy change (ΔG°) refers to the change in Gibbs Free Energy when reactants in their standard states are converted to products in their standard states at a specified temperature (usually 298 K). Standard states are defined as:
- For gases: 1 atmosphere pressure
- For liquids and solids: Pure substance at 1 atmosphere pressure
- For solutions: 1 molar concentration
Standard Gibbs Free Energy changes are tabulated for many reactions and can be used to calculate the ΔG° for other reactions using Hess's Law. This law allows us to add the ΔG° values of several reactions to determine the ΔG° of a target reaction by manipulating and combining the given equations.
Factors Affecting Gibbs Free Energy
Several factors can influence the Gibbs Free Energy of a reaction:
-
Temperature: Temperature's effect depends on the sign of ΔS. Increasing temperature favors reactions with positive ΔS, while decreasing temperature favors reactions with negative ΔS.
-
Pressure: Changes in pressure primarily affect reactions involving gases. Increased pressure favors reactions that result in a decrease in the number of gas molecules.
-
Concentration: The concentration of reactants and products directly influences the Gibbs Free Energy. Higher reactant concentrations favor the forward reaction, while higher product concentrations favor the reverse reaction. This is captured by the relationship between ΔG and the reaction quotient (Q). This relationship is given by:
ΔG = ΔG° + RTlnQ
Where Q is the reaction quotient, which represents the ratio of products to reactants at any given time during the reaction.
Gibbs Free Energy and Non-Standard Conditions
While ΔG° provides valuable information under standard conditions, real-world reactions rarely occur under standard conditions. The equation incorporating the reaction quotient (Q) is crucial for determining the Gibbs Free Energy under non-standard conditions. When Q is less than K (the equilibrium constant), ΔG is negative, indicating that the forward reaction is spontaneous. When Q is greater than K, ΔG is positive, indicating that the reverse reaction is spontaneous. When Q equals K, ΔG is zero, indicating that the system is at equilibrium.
Conclusion: The Power of Negative Gibbs Free Energy
Understanding the significance of negative Gibbs Free Energy is paramount in various scientific disciplines. It allows us to predict the spontaneity of reactions, determine the equilibrium position, and interpret the thermodynamic driving forces behind countless chemical and physical processes. From everyday phenomena to complex biochemical pathways, the concept provides a powerful framework for analyzing and understanding the world around us. The relationship between Gibbs Free Energy, enthalpy, entropy, and temperature provides a complete picture of the spontaneity and feasibility of chemical and physical transformations. Mastering this concept is essential for anyone pursuing a deeper understanding of thermodynamics and its applications.
Latest Posts
Latest Posts
-
What Determines The Shape Of A Molecule
Apr 18, 2025
-
20 Out Of 80 Is What Percent
Apr 18, 2025
-
How Many Valence Electrons Does Kr Have
Apr 18, 2025
-
Sequence Of Events In Cellular Respiration
Apr 18, 2025
-
Compare And Contrast Sound Waves And Electromagnetic Waves
Apr 18, 2025
Related Post
Thank you for visiting our website which covers about What Does Negative Gibbs Free Energy Mean . We hope the information provided has been useful to you. Feel free to contact us if you have any questions or need further assistance. See you next time and don't miss to bookmark.