What Does It Mean That Neurons Are Excitable
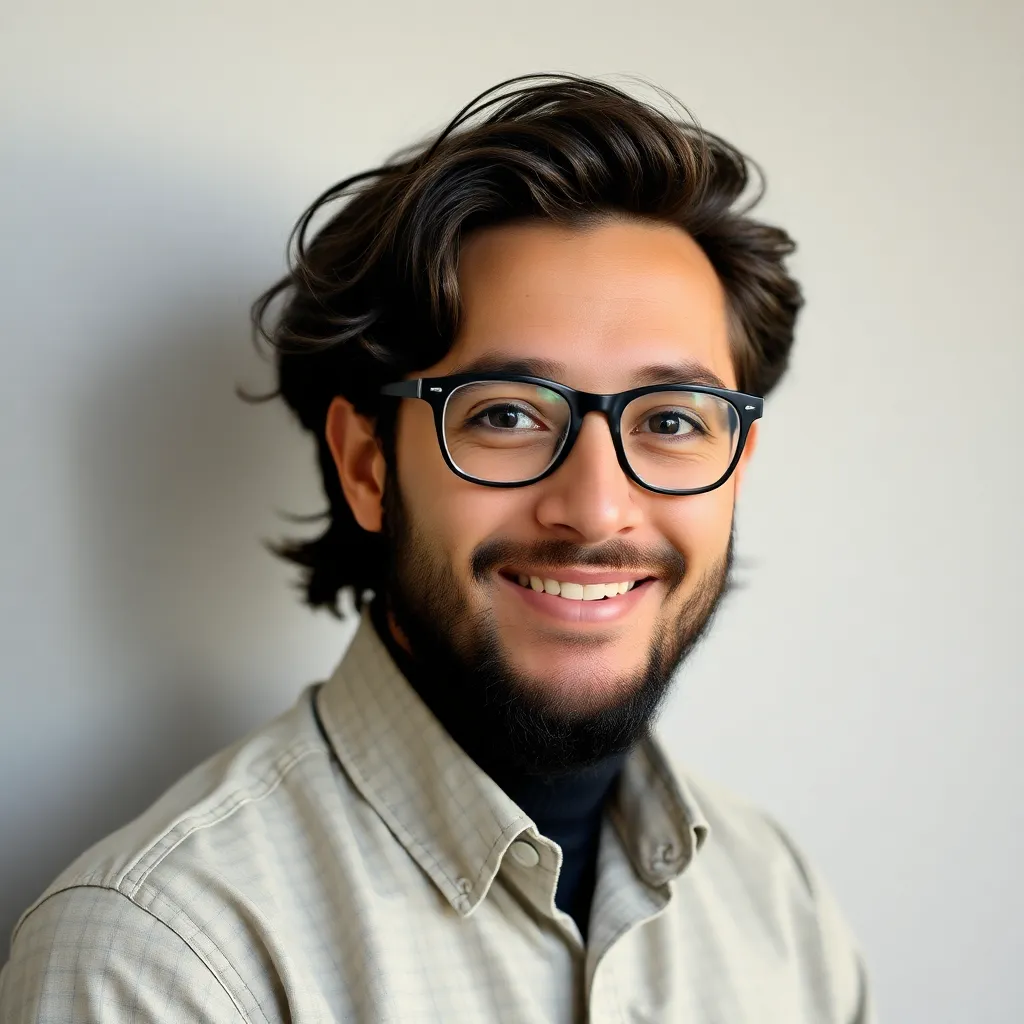
listenit
May 27, 2025 · 6 min read
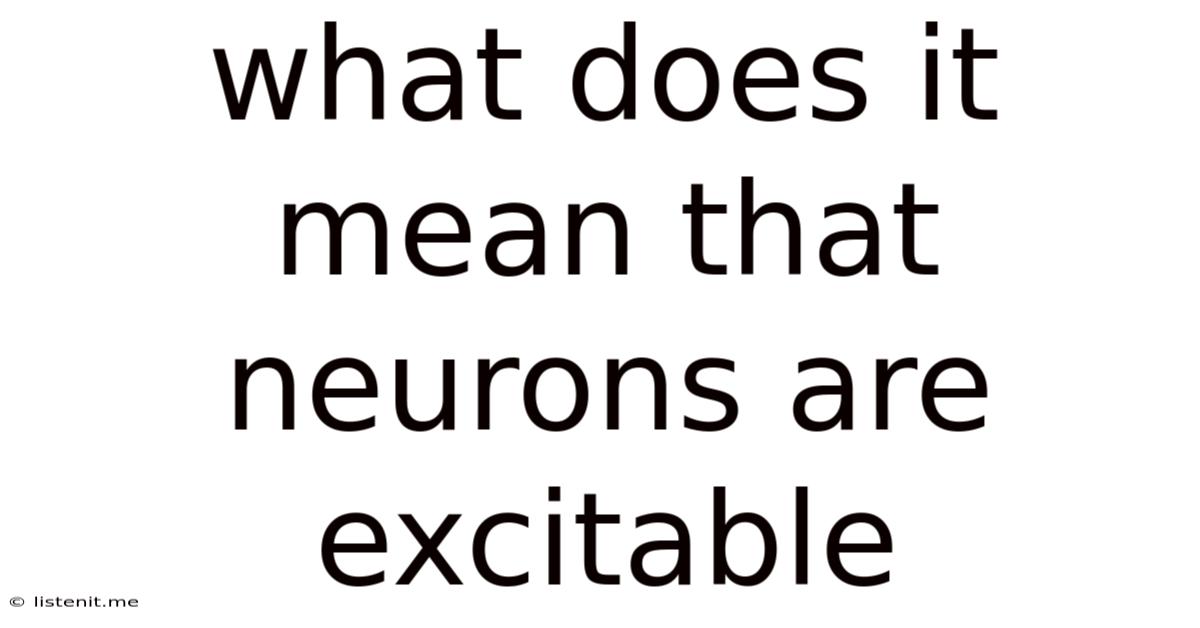
Table of Contents
What Does it Mean That Neurons are Excitable?
Neurons, the fundamental units of the nervous system, are remarkable cells possessing a unique and crucial property: excitability. This characteristic allows them to rapidly respond to stimuli, generating electrical signals that form the basis of all neural communication. Understanding neuronal excitability is paramount to comprehending how the brain functions, how we perceive the world, and how our bodies respond to internal and external cues. This article delves deep into the meaning of neuronal excitability, exploring the underlying mechanisms, its significance in neural signaling, and its implications for various neurological processes and disorders.
The Electrochemical Nature of Neuronal Excitability
The excitability of neurons stems from their unique electrochemical properties. Unlike other cells, neurons maintain a significant difference in electrical potential across their cell membrane, a phenomenon known as the resting membrane potential. This potential, typically around -70 millivolts (mV), is negative inside relative to the outside of the neuron. This resting potential is meticulously maintained by a complex interplay of ion channels and pumps embedded within the neuronal membrane.
The Role of Ion Channels and Pumps
-
Ion Channels: These are protein pores that selectively allow certain ions (like sodium (Na+), potassium (K+), chloride (Cl-), and calcium (Ca2+)) to pass across the membrane. Crucially, these channels are not always open; many are voltage-gated, meaning they open or close in response to changes in membrane potential. Others are ligand-gated, opening in response to the binding of specific neurotransmitters. This regulated permeability is the cornerstone of neuronal excitability.
-
Ion Pumps: These are protein complexes that actively transport ions against their concentration gradients, using energy from ATP hydrolysis. The most important of these is the sodium-potassium pump (Na+/K+ ATPase), which maintains the resting membrane potential by pumping three Na+ ions out of the cell for every two K+ ions pumped in. This creates a net negative charge inside the neuron.
The Action Potential: The Neuron's Electrical Signal
When a neuron receives sufficient stimulation, its membrane potential depolarizes—it becomes less negative. This depolarization occurs when ion channels open, allowing positively charged ions, predominantly Na+, to rush into the cell. If this depolarization reaches a critical threshold (typically around -55 mV), it triggers an action potential.
Stages of the Action Potential
The action potential is a rapid, transient reversal of the membrane potential, a self-propagating electrical signal that travels down the axon, the neuron's long projection. It unfolds in several key stages:
-
Depolarization: Voltage-gated Na+ channels open rapidly, causing a massive influx of Na+ ions and a sharp rise in membrane potential.
-
Repolarization: Na+ channels inactivate, and voltage-gated K+ channels open, allowing K+ ions to flow out of the cell, restoring the negative membrane potential.
-
Hyperpolarization: K+ channels remain open slightly longer than needed, causing a temporary hyperpolarization (membrane potential becomes more negative than the resting potential).
-
Return to Resting Potential: The Na+/K+ pump and ion leakage gradually restore the resting membrane potential.
The action potential is an "all-or-nothing" event: either it occurs fully, or it doesn't. The intensity of the stimulus doesn't affect the amplitude of the action potential; instead, it's encoded in the frequency of action potentials—a stronger stimulus generates a higher frequency of action potentials.
Propagation of the Action Potential
The action potential doesn't passively decay as it travels down the axon. Instead, it's actively propagated through a process of continuous or saltatory conduction.
-
Continuous Conduction: In unmyelinated axons, the action potential regenerates repeatedly along the entire length of the axon, a slower process.
-
Saltatory Conduction: In myelinated axons (axons covered in myelin sheaths), the action potential "jumps" between the Nodes of Ranvier, the gaps in the myelin sheath. This significantly speeds up conduction velocity. Myelin acts as an insulator, preventing ion leakage and allowing for efficient propagation.
Neuronal Excitability and Synaptic Transmission
The action potential reaches the axon terminal, where it triggers the release of neurotransmitters. These chemical messengers are crucial for communication between neurons at specialized junctions called synapses.
Synaptic Transmission: Excitatory and Inhibitory Signals
Neurotransmitters bind to receptors on the postsynaptic neuron, inducing either excitatory or inhibitory postsynaptic potentials (EPSPs and IPSPs).
-
EPSPs: These depolarize the postsynaptic neuron, bringing its membrane potential closer to the threshold for action potential generation. They are typically mediated by neurotransmitters like glutamate.
-
IPSPs: These hyperpolarize the postsynaptic neuron, moving its membrane potential further away from the threshold. They are often mediated by neurotransmitters like GABA (gamma-aminobutyric acid).
The postsynaptic neuron integrates these EPSPs and IPSPs. If the sum of excitatory inputs surpasses the threshold, an action potential is generated in the postsynaptic neuron. This summation of excitatory and inhibitory signals is a fundamental mechanism for information processing in the nervous system.
Modulation of Neuronal Excitability
Neuronal excitability isn't static; it can be modulated by various factors, including:
-
Neurotransmitters: Beyond their role in synaptic transmission, some neurotransmitters can directly influence the excitability of neurons by affecting the properties of ion channels.
-
Neuromodulators: These substances, often released diffusely, can alter the sensitivity of neurons to other neurotransmitters or directly modify the properties of ion channels, influencing the overall excitability of neuronal circuits.
-
Hormones: Endocrine signals can exert long-lasting effects on neuronal excitability, playing a role in processes like stress responses, mood regulation, and learning.
-
Drugs: Many drugs act by influencing neuronal excitability, either by enhancing or suppressing the activity of specific ion channels or receptors. This is the basis of the action of many psychoactive substances.
Implications of Neuronal Excitability for Neurological Disorders
Disruptions in neuronal excitability are implicated in a wide range of neurological disorders:
-
Epilepsy: Characterized by excessive and synchronous neuronal activity, leading to seizures. This often involves imbalances in excitatory and inhibitory neurotransmission.
-
Stroke: Damage to brain tissue due to impaired blood supply can alter neuronal excitability, leading to neuronal death and neurological deficits.
-
Multiple Sclerosis (MS): Demyelination of axons impairs saltatory conduction, disrupting neuronal signaling.
-
Neurodegenerative Diseases (Alzheimer's, Parkinson's): These conditions often involve progressive changes in neuronal excitability, contributing to cognitive decline and motor dysfunction.
Conclusion: A Complex and Dynamic Process
Neuronal excitability is a fundamental process enabling the nervous system to function. This intricate interplay of ion channels, pumps, neurotransmitters, and neuromodulators allows for rapid and adaptable communication, forming the foundation for all aspects of brain function, from sensory perception and motor control to cognition and emotion. Understanding the complexities of neuronal excitability is essential for unraveling the mechanisms underlying both normal brain function and a wide array of neurological disorders. Further research into this area is crucial for developing effective therapeutic strategies for neurological conditions and enhancing our understanding of the brain's remarkable capabilities. The dynamic nature of neuronal excitability and its susceptibility to modulation provide a rich area of study with far-reaching implications for neuroscience and medicine. Continued research will undoubtedly reveal even more nuanced aspects of this crucial cellular property.
Latest Posts
Latest Posts
-
Ip De La Arteria Cerebral Media
May 28, 2025
-
Socioeconomic Status Refers To An Individuals
May 28, 2025
-
How To Find The Fundamental Period
May 28, 2025
-
How Do You Tell How Old A Palm Tree Is
May 28, 2025
-
A Homogeneous Linear System Is Always Consistent
May 28, 2025
Related Post
Thank you for visiting our website which covers about What Does It Mean That Neurons Are Excitable . We hope the information provided has been useful to you. Feel free to contact us if you have any questions or need further assistance. See you next time and don't miss to bookmark.