What Determines The Final Shape Of The Protein Molecule
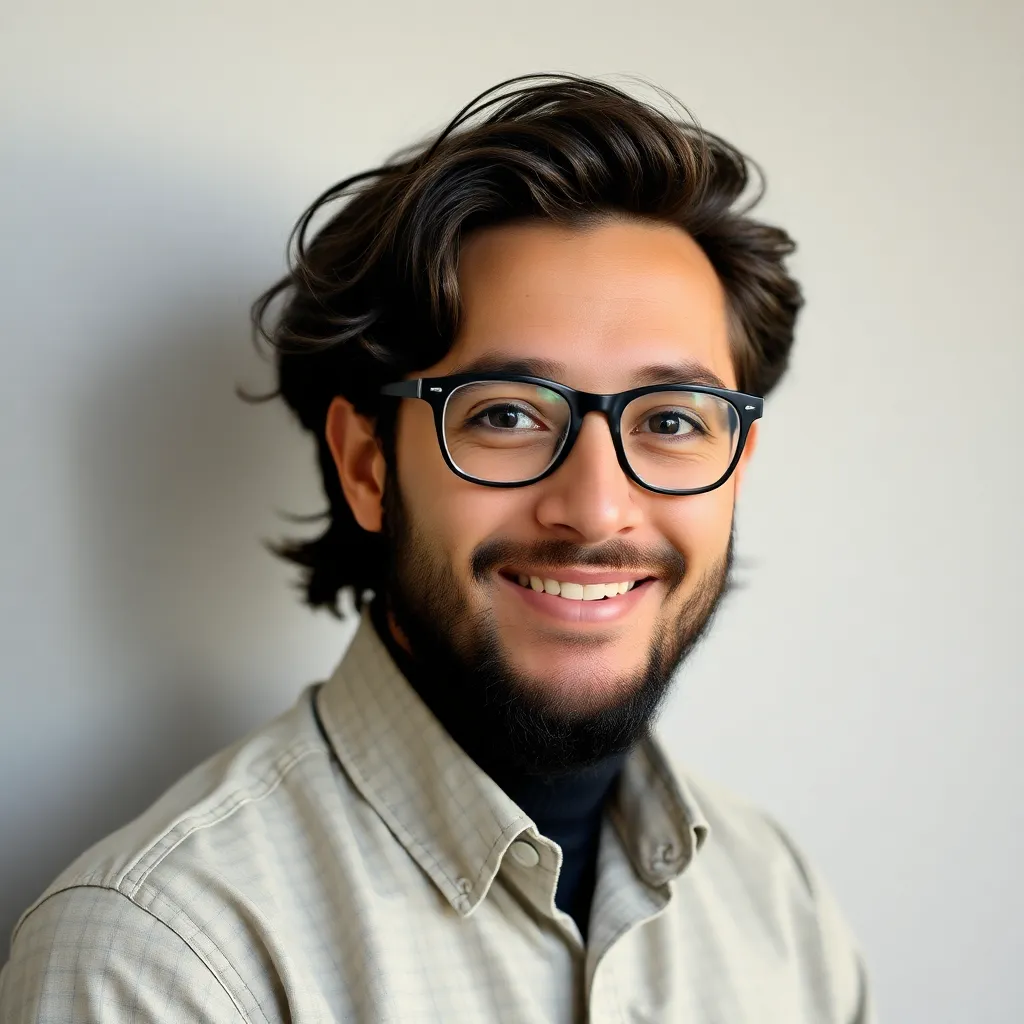
listenit
Apr 23, 2025 · 6 min read

Table of Contents
What Determines the Final Shape of a Protein Molecule?
Proteins are the workhorses of the cell, carrying out a vast array of functions crucial for life. Their ability to perform these diverse roles hinges critically on their three-dimensional structure, a complex shape determined by a fascinating interplay of factors. Understanding how a protein folds into its final, functional conformation is a fundamental challenge in biology, with implications for medicine, biotechnology, and our understanding of life itself.
The Primary Structure: The Blueprint of the Protein
The journey to a protein's final shape begins with its primary structure: the linear sequence of amino acids. This sequence is dictated by the gene encoding the protein, a precise instruction manual written in the language of DNA. Each amino acid possesses unique chemical properties—some are hydrophobic (water-fearing), others hydrophilic (water-loving), some are positively charged, others negatively charged, and some have bulky side chains while others are small. This inherent chemical diversity is the foundation upon which the protein's higher-order structures are built. Even a single amino acid substitution can dramatically alter the final shape and function of the protein, as tragically illustrated in diseases like sickle cell anemia, caused by a single point mutation in the hemoglobin gene.
The Importance of Peptide Bonds
The amino acids are linked together by peptide bonds, strong covalent bonds that form the backbone of the polypeptide chain. The peptide bond itself has a partial double bond character, which restricts rotation around the bond and contributes to the overall rigidity of the polypeptide chain. This rigidity is important in defining the local conformations of the protein.
Secondary Structure: Local Folding Patterns
The primary sequence, with its inherent chemical properties and the constraints imposed by peptide bonds, begins to fold into local structural motifs known as secondary structures. These are stabilized by hydrogen bonds formed between the backbone atoms of the polypeptide chain. Two dominant types of secondary structure are:
Alpha-Helices
Alpha-helices are coiled structures stabilized by hydrogen bonds between the carbonyl oxygen of one amino acid and the amide hydrogen of the amino acid four residues down the chain. The side chains of the amino acids extend outwards from the helix. The stability of an alpha-helix is influenced by the size and charge of the side chains. Certain amino acids, like proline, disrupt alpha-helix formation due to their rigid structure.
Beta-Sheets
Beta-sheets are formed by extended stretches of polypeptide chains arranged side-by-side. Hydrogen bonds form between the carbonyl oxygen and amide hydrogen of adjacent strands. Beta-sheets can be parallel (strands running in the same direction) or antiparallel (strands running in opposite directions). The side chains of amino acids in beta-sheets alternate above and below the plane of the sheet.
Loops and Turns
In addition to alpha-helices and beta-sheets, proteins also contain regions of irregular structure called loops and turns. These regions are often located on the protein surface and are involved in interactions with other molecules. They are crucial for protein function because they often form binding sites for ligands or other proteins.
Tertiary Structure: The Overall 3D Arrangement
The arrangement of secondary structure elements in three-dimensional space constitutes the tertiary structure. This is where the protein truly takes on its unique shape, dictated by a complex interplay of various forces:
Hydrophobic Interactions
One of the most dominant forces driving tertiary structure is the hydrophobic effect. Hydrophobic amino acid side chains tend to cluster together in the protein's interior, away from the aqueous environment, minimizing their contact with water. This creates a hydrophobic core, a crucial element in protein stability.
Hydrogen Bonds
Hydrogen bonds play a significant role in tertiary structure, forming between various side chains and backbone atoms. These bonds help to stabilize the folded conformation of the protein.
Ionic Bonds (Salt Bridges)
Ionic interactions, or salt bridges, occur between oppositely charged amino acid side chains. These electrostatic interactions contribute to protein stability, particularly on the protein surface.
Disulfide Bonds
Disulfide bonds, covalent bonds formed between the sulfur atoms of cysteine residues, are particularly strong and contribute significantly to the stability of some proteins. These bonds often cross-link different parts of the protein, reinforcing the overall structure.
Quaternary Structure: The Assembly of Subunits
Many proteins consist of multiple polypeptide chains, called subunits, that assemble together to form a functional protein complex. This arrangement is known as the quaternary structure. The same forces that govern tertiary structure—hydrophobic interactions, hydrogen bonds, ionic bonds, and disulfide bonds—also mediate the interactions between subunits. The precise arrangement of subunits is critical for the protein's function. Hemoglobin, for example, a tetramer composed of four subunits, exemplifies the importance of quaternary structure.
Factors Influencing Protein Folding
The final shape of a protein is not simply a deterministic consequence of its primary sequence. Several other factors influence the folding process:
Molecular Chaperones
Molecular chaperones are proteins that assist other proteins in folding correctly. They prevent aggregation and misfolding, ensuring that proteins reach their native conformation efficiently. Chaperonins, a specific class of chaperones, provide a protected environment for protein folding.
Post-Translational Modifications
Post-translational modifications (PTMs), such as glycosylation, phosphorylation, and ubiquitination, can significantly alter a protein's shape and function. These modifications often occur after the protein has been synthesized and folded, affecting its interaction with other molecules.
Environmental Factors
The cellular environment plays a crucial role in protein folding. Factors such as temperature, pH, and ionic strength can influence the stability of protein structure. Extreme changes in these parameters can lead to protein denaturation, where the protein unfolds and loses its function.
Protein Misfolding and Diseases
When proteins fail to fold correctly, they can lead to a range of diseases, collectively known as protein misfolding diseases. These include neurodegenerative diseases such as Alzheimer's and Parkinson's, where misfolded proteins aggregate to form amyloid fibrils. Understanding the mechanisms of protein misfolding is crucial for developing effective therapies for these devastating conditions.
Computational Approaches to Protein Structure Prediction
Predicting the three-dimensional structure of a protein from its amino acid sequence remains a major challenge in bioinformatics. However, recent advances in computational methods, particularly deep learning-based approaches like AlphaFold, have revolutionized the field, achieving remarkable accuracy in predicting protein structures. These methods leverage vast datasets of known protein structures to predict the structures of novel proteins, opening up exciting possibilities for drug discovery and understanding biological processes.
Conclusion
The final shape of a protein molecule is a remarkable feat of biological engineering, a consequence of the precise interplay between its amino acid sequence, various non-covalent forces, chaperones, post-translational modifications, and the cellular environment. This intricate process is essential for the protein's function, and disruptions in this process can have profound consequences for human health. Continued research in this area promises to unravel further the mysteries of protein folding, paving the way for advances in medicine and biotechnology. The journey from a linear sequence of amino acids to a functional three-dimensional structure is a testament to the elegance and complexity of life itself. Understanding this journey is vital for addressing numerous biological and medical challenges, furthering our knowledge of protein function and disease. The remarkable progress made in recent years, particularly with computational approaches, offers hope for even greater breakthroughs in the future.
Latest Posts
Latest Posts
-
Simplify The Square Root Of 160
Apr 24, 2025
-
What Is One Half Of Two Thirds
Apr 24, 2025
-
Equation Of Parabola With Vertex And Focus
Apr 24, 2025
-
What Is 6 To The Zero Power
Apr 24, 2025
-
What Is A Factor Of 44
Apr 24, 2025
Related Post
Thank you for visiting our website which covers about What Determines The Final Shape Of The Protein Molecule . We hope the information provided has been useful to you. Feel free to contact us if you have any questions or need further assistance. See you next time and don't miss to bookmark.