What Biomolecule Are Enzymes Made Of
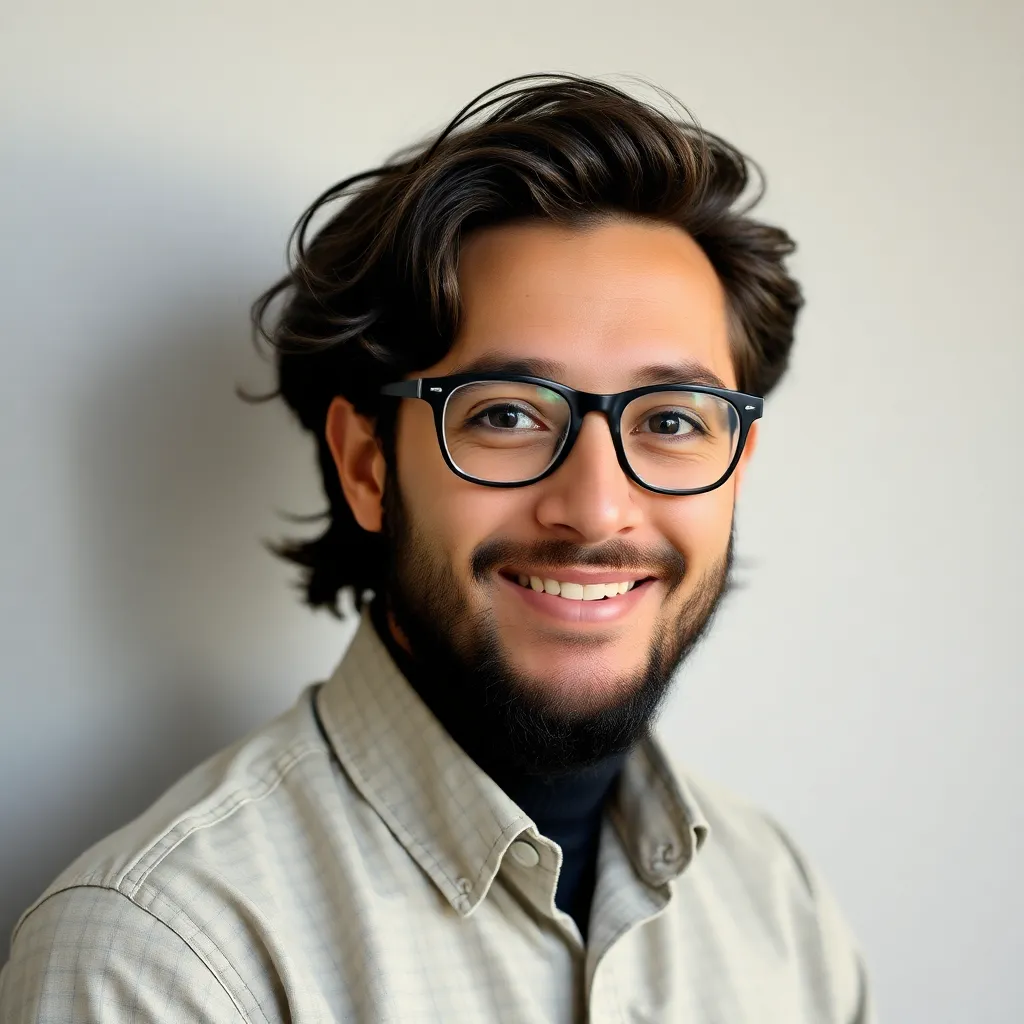
listenit
Apr 08, 2025 · 6 min read

Table of Contents
What Biomolecule Are Enzymes Made Of?
Enzymes are the workhorses of life, orchestrating countless biochemical reactions within living organisms. Understanding their composition is crucial to grasping their function and the intricate mechanisms they drive. The short answer is: enzymes are made of proteins, but this simple statement belies the complex and fascinating world of enzymatic structure and function. This article delves deep into the biomolecular composition of enzymes, exploring their protein nature, the role of cofactors and coenzymes, and the impact of their structure on their catalytic activity.
The Predominant Building Block: Proteins
The vast majority of enzymes are proteins. Proteins are polymers of amino acids, linked together by peptide bonds to form polypeptide chains. The sequence of amino acids, known as the primary structure, dictates the enzyme's three-dimensional shape. This three-dimensional structure, encompassing secondary, tertiary, and sometimes quaternary levels, is absolutely crucial for enzyme function.
Levels of Protein Structure and Enzymatic Activity
-
Primary Structure: This refers to the linear sequence of amino acids in the polypeptide chain. The specific order of amino acids is genetically encoded and determines all subsequent levels of structure. Even a single amino acid change can drastically alter enzyme activity, as seen in sickle cell anemia, caused by a single amino acid substitution in hemoglobin.
-
Secondary Structure: This level describes local folding patterns within the polypeptide chain, primarily alpha-helices and beta-sheets. These structures are stabilized by hydrogen bonds between the amino acid backbones. The arrangement of alpha-helices and beta-sheets contributes significantly to the enzyme's overall shape and creates pockets or grooves where substrates bind.
-
Tertiary Structure: This represents the overall three-dimensional arrangement of the polypeptide chain, including the spatial relationships between secondary structure elements. Tertiary structure is stabilized by various interactions, including disulfide bonds, hydrophobic interactions, ionic bonds, and hydrogen bonds between side chains of amino acids. This intricate folding creates the active site, the region of the enzyme where the substrate binds and the catalytic reaction takes place.
-
Quaternary Structure: Some enzymes consist of multiple polypeptide chains (subunits) assembled together. This arrangement is the quaternary structure. The interactions between subunits, similar to those stabilizing tertiary structure, are crucial for maintaining the enzyme's overall shape and function. Many enzymes exhibiting allosteric regulation possess quaternary structure.
The precise folding of the protein into its specific three-dimensional conformation is crucial for enzyme activity. Any disruption to this structure, caused by factors like heat, pH changes, or the presence of denaturants, can lead to denaturation, resulting in loss of enzymatic activity. This highlights the intimate connection between an enzyme's protein structure and its catalytic function.
Beyond Proteins: Cofactors and Coenzymes
While proteins form the backbone of most enzymes, many require additional non-protein components called cofactors to function properly. These cofactors can be inorganic ions (like metal ions such as zinc, iron, magnesium, or copper) or organic molecules called coenzymes.
The Role of Cofactors
Inorganic cofactors often participate directly in the catalytic mechanism. For instance, zinc ions in carbonic anhydrase stabilize the active site and facilitate the reaction. Iron ions in cytochromes play a critical role in electron transport chains. These metal ions can act as electron acceptors or donors, facilitating redox reactions. The presence or absence of these cofactors significantly impacts the enzyme’s catalytic efficiency.
The Importance of Coenzymes
Coenzymes are organic molecules that often act as transient carriers of electrons, atoms, or functional groups during the enzymatic reaction. Many coenzymes are derived from vitamins. For example:
- Nicotinamide adenine dinucleotide (NAD+): A crucial coenzyme involved in redox reactions, derived from niacin (vitamin B3).
- Flavin adenine dinucleotide (FAD): Another coenzyme involved in redox reactions, derived from riboflavin (vitamin B2).
- Coenzyme A (CoA): Plays a central role in acyl group transfer reactions, essential in metabolism.
- Pyridoxal phosphate (PLP): Derived from vitamin B6, crucial for amino acid metabolism.
- Tetrahydrofolic acid (THF): Derived from folic acid, involved in one-carbon metabolism.
These coenzymes temporarily bind to the enzyme, participate in the catalytic process, and then dissociate, leaving the enzyme free to catalyze another reaction. The deficiency of certain vitamins can lead to enzyme dysfunction due to a lack of the corresponding coenzymes.
Enzyme Classification and Examples
Enzymes are classified into six major classes based on the type of reaction they catalyze:
- Oxidoreductases: Catalyze oxidation-reduction reactions. Examples include dehydrogenases and oxidases.
- Transferases: Catalyze the transfer of functional groups from one molecule to another. Examples include kinases and transaminases.
- Hydrolases: Catalyze hydrolysis reactions (breaking bonds using water). Examples include lipases and proteases.
- Lyases: Catalyze the addition or removal of groups to or from a double bond. Examples include decarboxylases and aldolases.
- Isomerases: Catalyze isomerization reactions (conversion of one isomer to another). Examples include mutases and epimerases.
- Ligases: Catalyze the joining of two molecules with the concomitant hydrolysis of ATP. Examples include DNA ligase and synthetase.
Each class contains numerous enzymes with diverse substrate specificities. The remarkable diversity in enzyme function stems from the immense variety of protein structures and the specific combination of amino acids within their active sites.
The Active Site: The Heart of Enzymatic Catalysis
The active site is a crucial region of the enzyme, a three-dimensional cleft or pocket where the substrate binds and the catalytic reaction occurs. The active site is typically composed of a small number of amino acid residues, strategically positioned to interact with the substrate. These interactions can be:
- Hydrogen bonds: Weak interactions between polar groups.
- Ionic bonds: Electrostatic interactions between charged groups.
- Hydrophobic interactions: Interactions between nonpolar groups.
- Covalent bonds: Stronger interactions formed during the reaction.
The active site's precise geometry and chemical properties determine the enzyme's specificity for its substrate. The "lock and key" model, though simplistic, illustrates the complementary shape between enzyme and substrate. The "induced fit" model provides a more realistic depiction, suggesting that the enzyme's shape changes upon substrate binding, optimizing interactions for catalysis.
Factors Affecting Enzyme Activity
Several factors can influence the rate of enzyme-catalyzed reactions:
-
Temperature: Enzymes generally have an optimal temperature at which they function most efficiently. Higher temperatures can denature the enzyme, while lower temperatures reduce the rate of reaction.
-
pH: Each enzyme has an optimal pH range. Changes in pH can alter the ionization state of amino acid residues, affecting the enzyme's structure and activity.
-
Substrate concentration: At low substrate concentrations, the rate of reaction is directly proportional to substrate concentration. However, at high substrate concentrations, the rate of reaction plateaus as the enzyme becomes saturated.
-
Enzyme concentration: The rate of reaction is directly proportional to enzyme concentration, provided that substrate is not limiting.
-
Inhibitors: Substances that reduce or inhibit enzyme activity are called inhibitors. They can be competitive (competing with the substrate for the active site) or non-competitive (binding to a different site, altering the enzyme's shape).
Conclusion: The Intricate World of Enzyme Structure and Function
Enzymes are remarkable biomolecules, predominantly composed of proteins, that drive the vast majority of biochemical reactions within living organisms. Their precise three-dimensional structure, determined by the amino acid sequence and stabilized by various interactions, is crucial for their catalytic activity. Many enzymes require additional non-protein components, such as cofactors and coenzymes, for optimal function. Understanding the biomolecular composition of enzymes, their structure-function relationship, and the factors affecting their activity is fundamental to comprehending the intricacies of life's processes. Further research continues to unveil the complexities of enzyme mechanisms and their roles in various biological pathways, promising exciting advancements in fields such as medicine, biotechnology, and environmental science.
Latest Posts
Latest Posts
-
How Many Gallons Is 30 Litres
Apr 16, 2025
-
6 Is 75 Of What Number
Apr 16, 2025
-
Greatest Common Factor Of 56 And 42
Apr 16, 2025
-
The Function Of Cellular Respiration Is To
Apr 16, 2025
-
How Many Meters Are In One Light Year
Apr 16, 2025
Related Post
Thank you for visiting our website which covers about What Biomolecule Are Enzymes Made Of . We hope the information provided has been useful to you. Feel free to contact us if you have any questions or need further assistance. See you next time and don't miss to bookmark.