Transfer Of Heat Through Electromagnetic Waves
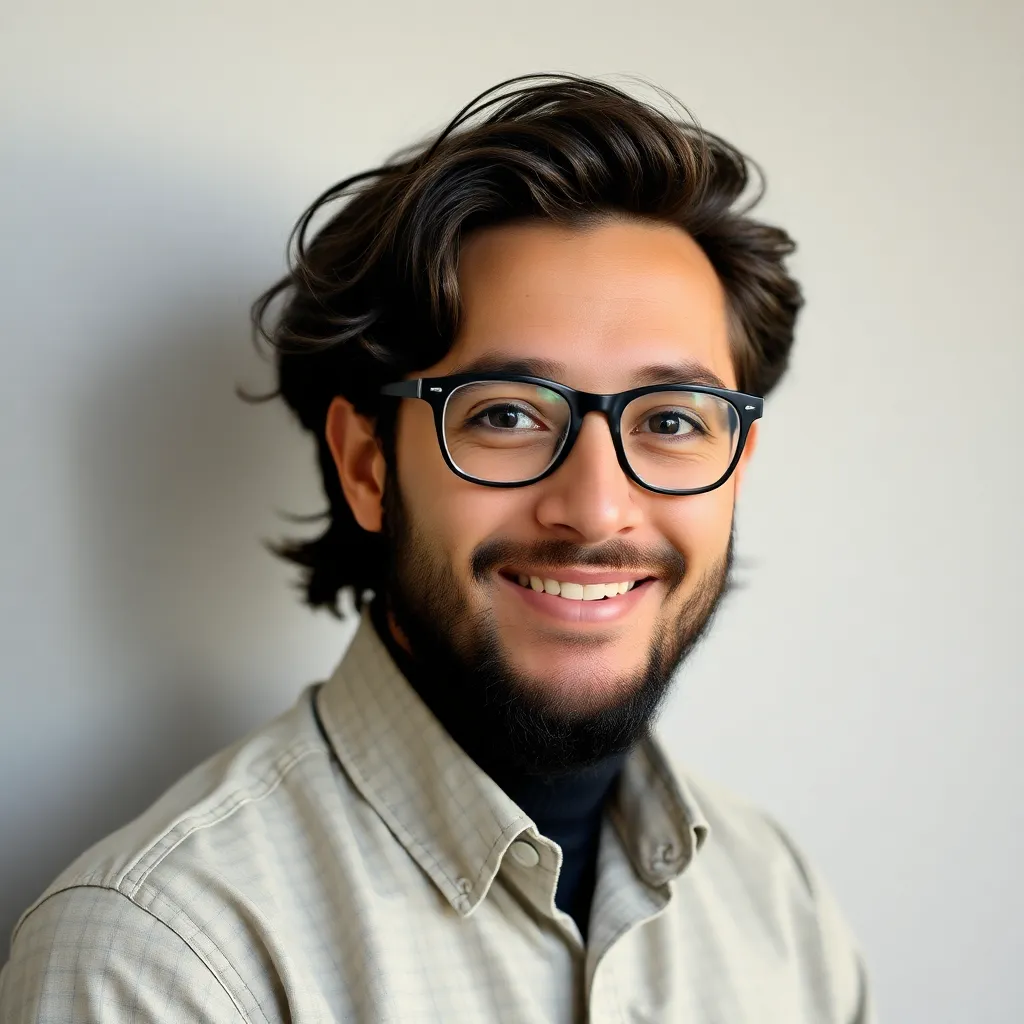
listenit
May 10, 2025 · 7 min read
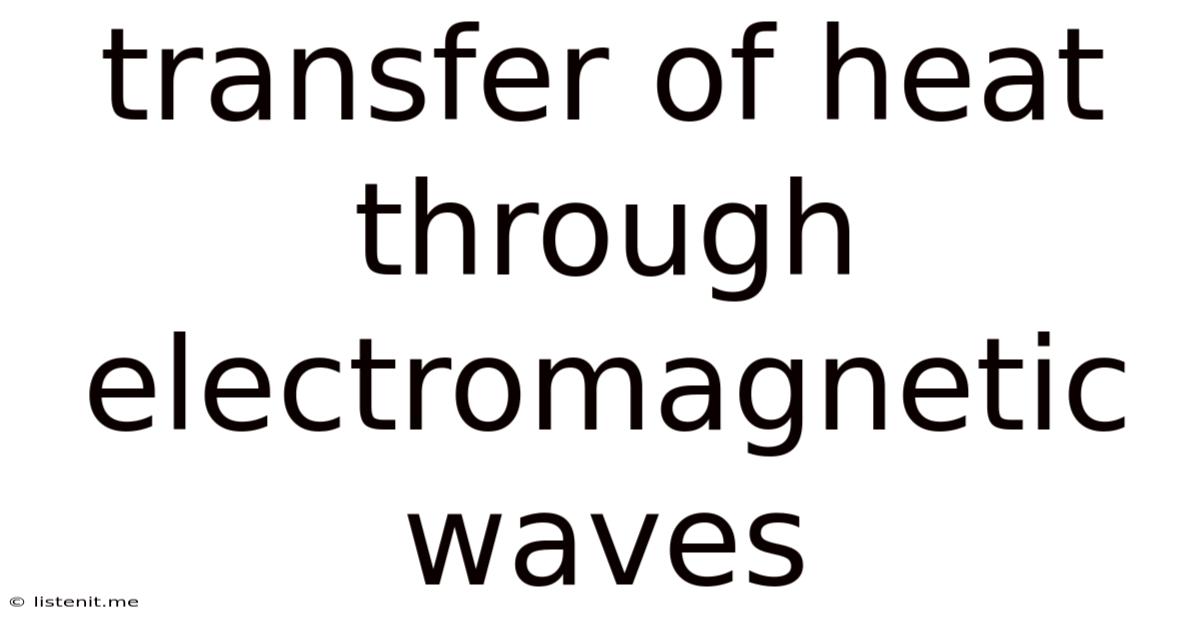
Table of Contents
Transfer of Heat Through Electromagnetic Waves: A Deep Dive into Radiation
Heat transfer, a fundamental concept in physics and engineering, encompasses three primary mechanisms: conduction, convection, and radiation. While conduction relies on direct contact between molecules, and convection involves the movement of fluids, radiation stands apart, transferring heat through electromagnetic waves. This article delves deep into the fascinating world of radiative heat transfer, exploring its principles, applications, and significance.
Understanding Electromagnetic Waves and Heat Transfer
Electromagnetic waves, a form of energy propagation, encompass a broad spectrum, ranging from radio waves to gamma rays. Visible light occupies a small portion of this spectrum, but all electromagnetic waves carry energy. Thermal radiation, specifically, is electromagnetic radiation generated by the thermal motion of charged particles within matter. The hotter an object, the more intense its thermal radiation. This radiation travels at the speed of light and doesn't require a medium; it can traverse the vacuum of space, unlike conduction and convection.
The Electromagnetic Spectrum and Heat Transfer
The relationship between wavelength and the amount of energy carried by electromagnetic waves is crucial. Shorter wavelengths, like those of ultraviolet (UV) and X-rays, carry significantly more energy than longer wavelengths, such as infrared (IR) and radio waves. While the entire electromagnetic spectrum contributes to radiative heat transfer, the infrared region is particularly important in everyday scenarios. Most of the heat we feel from the sun, a fire, or a radiator is in the form of infrared radiation.
Blackbody Radiation: A Theoretical Ideal
A blackbody is a theoretical object that absorbs all incident electromagnetic radiation, regardless of wavelength or angle. While perfect blackbodies don't exist in nature, many objects approximate this behavior. A blackbody also emits radiation, and the intensity and spectral distribution of this emitted radiation depend solely on its temperature. This relationship is described by Planck's Law, a fundamental equation in physics that provides the spectral radiance of a blackbody as a function of temperature and wavelength.
Planck's Law allows us to understand the spectral distribution of blackbody radiation, which shows that hotter objects emit more radiation at all wavelengths and peak at shorter wavelengths. This explains why objects glow red-hot at lower temperatures and become white-hot at higher temperatures. The total power radiated per unit area by a blackbody is given by the Stefan-Boltzmann Law, which states that the power is proportional to the fourth power of its absolute temperature. This significant dependence on temperature highlights the importance of temperature in radiative heat transfer.
Factors Affecting Radiative Heat Transfer
Several factors influence the rate at which heat is transferred through radiation:
1. Temperature Difference: The Driving Force
The temperature difference between the emitting and absorbing surfaces is the primary driving force behind radiative heat transfer. A larger temperature difference results in a higher rate of heat transfer. This is directly reflected in the Stefan-Boltzmann Law, which shows a strong dependence on the fourth power of temperature.
2. Emissivity: A Measure of Radiation Efficiency
Emissivity (ε) is a crucial property of a material that describes its effectiveness in emitting thermal radiation. It ranges from 0 to 1, with 1 representing a perfect blackbody. A higher emissivity indicates a more efficient emitter, meaning more heat is radiated away. Conversely, a lower emissivity indicates a more reflective surface, reducing the amount of heat radiated. For example, polished metals have low emissivity, while matte surfaces have higher emissivity.
3. Absorptivity: The Ability to Absorb Radiation
Absorptivity (α) describes the fraction of incident radiation absorbed by a surface. Similar to emissivity, it ranges from 0 to 1. A high absorptivity means that the surface readily absorbs incoming radiation, while a low absorptivity means it reflects most of the radiation. Kirchhoff's Law of Thermal Radiation states that for an object in thermal equilibrium, its emissivity is equal to its absorptivity at a given wavelength and temperature. This means good emitters are also good absorbers.
4. Surface Area: The Size Matters
The surface area involved in the heat transfer process directly affects the rate of radiation. Larger surfaces radiate and absorb more heat than smaller surfaces. This is intuitively understandable: a larger area provides more opportunities for radiation to interact with the material.
5. Distance: The Inverse Square Law
The distance between the emitting and absorbing surfaces is crucial. Radiation intensity decreases with the square of the distance between the surfaces. This is known as the inverse square law. The further apart the surfaces are, the less effective the radiative heat transfer.
Applications of Radiative Heat Transfer
Radiative heat transfer plays a crucial role in numerous applications across various fields:
1. Space Exploration: Surviving the Vacuum
In space exploration, understanding radiative heat transfer is paramount. Spacecraft must be designed to withstand extreme temperature variations while maintaining operational temperatures. The vacuum of space precludes conduction and convection, making radiation the dominant mode of heat transfer. Specialized coatings and materials are used to control the absorption and emission of radiation, ensuring the spacecraft's survival.
2. Solar Energy: Harnessing the Sun's Power
Solar energy technologies rely heavily on radiative heat transfer. Solar panels absorb solar radiation and convert it into electricity. The design of solar collectors needs to optimize the absorption of solar radiation while minimizing heat losses through radiation, convection, and conduction.
3. Industrial Furnaces and Kilns: High-Temperature Processes
Industrial furnaces and kilns utilize radiative heat transfer extensively. The heating elements radiate heat onto the materials being processed, facilitating high-temperature applications like metal smelting and ceramic firing. The design and operation of these furnaces require a thorough understanding of radiative heat transfer to control temperature profiles efficiently.
4. Building Design: Passive Heating and Cooling
Building design incorporates radiative heat transfer principles to enhance energy efficiency. Features like strategically placed windows and thermal mass can leverage solar radiation for passive heating in winter and minimize heat gain in summer. The selection of building materials with appropriate emissivity and absorptivity is crucial for achieving thermal comfort and reducing energy consumption.
5. Medical Applications: Infrared Thermography
Infrared thermography employs the principles of radiative heat transfer to detect temperature variations on the surface of the body. This technique is valuable in medical diagnostics, allowing for the detection of inflammation, tumors, and other abnormalities based on their thermal signatures.
Advanced Concepts and Considerations
Beyond the fundamentals, understanding radiative heat transfer involves several advanced concepts:
1. View Factors: Geometric Considerations
View factors account for the geometric relationships between radiating surfaces. They represent the fraction of radiation leaving one surface that strikes another surface. Calculating view factors is crucial for accurate analysis in complex geometries, often requiring numerical methods.
2. Participating Media: Radiation within Gases
The analysis of radiative heat transfer becomes more complex when considering participating media, such as gases and aerosols. These media can absorb, emit, and scatter radiation, altering the radiative transfer process. The treatment of participating media often involves sophisticated numerical techniques and radiative transfer models.
3. Radiative Properties of Materials: Beyond Emissivity and Absorptivity
The radiative properties of materials, such as reflectivity, transmissivity, and scattering properties, are essential for accurate modeling of radiative heat transfer. These properties are wavelength-dependent and can vary significantly with temperature and surface conditions. Experimental measurements and advanced characterization techniques are crucial for obtaining accurate radiative property data.
Conclusion: The Ubiquity of Radiative Heat Transfer
Radiative heat transfer is a pervasive phenomenon with far-reaching implications across diverse scientific and engineering disciplines. Understanding its principles, influencing factors, and advanced concepts is vital for designing efficient and sustainable technologies, optimizing industrial processes, and advancing scientific knowledge. From the vastness of space to the intricacies of human physiology, radiation's role in heat transfer is undeniable, making its study both crucial and endlessly fascinating. Further research and development in areas such as advanced materials, numerical modeling, and radiative property characterization will continue to expand our understanding and applications of this fundamental mode of heat transfer.
Latest Posts
Latest Posts
-
Can A Plant Live Without Oxygen
May 10, 2025
-
How To Find The Area Of A Parallelogram With Vectors
May 10, 2025
-
Find The Radian Measure Of An Angle Of 110
May 10, 2025
-
Dna Is A Polymer Of Nucleotides
May 10, 2025
-
What Is The Greatest Common Factor Of 90
May 10, 2025
Related Post
Thank you for visiting our website which covers about Transfer Of Heat Through Electromagnetic Waves . We hope the information provided has been useful to you. Feel free to contact us if you have any questions or need further assistance. See you next time and don't miss to bookmark.