Three Parts Of An Atp Molecule
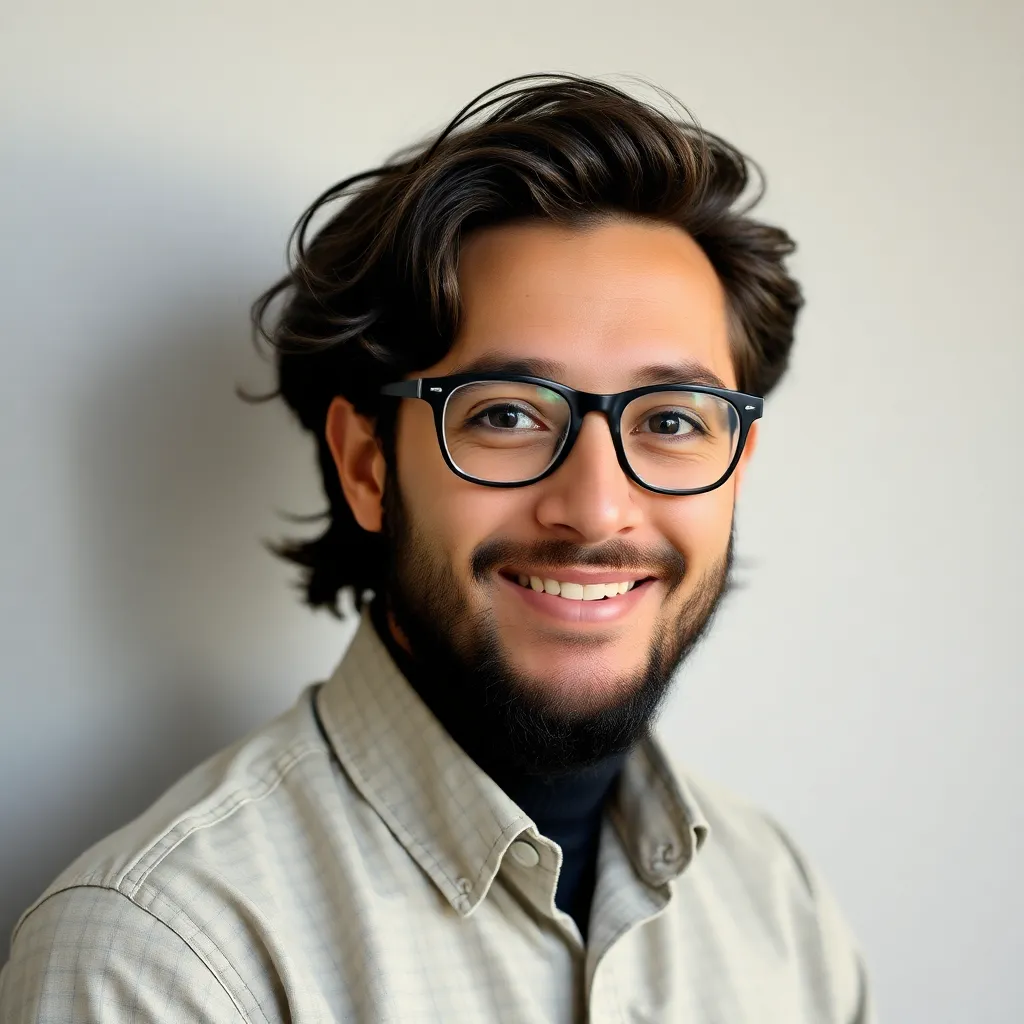
listenit
Apr 04, 2025 · 7 min read

Table of Contents
Decoding ATP: A Deep Dive into the Three Parts of the Energy Currency of Life
Adenosine triphosphate (ATP) – the very name conjures images of cellular energy. It’s the powerhouse molecule fueling virtually every biological process in living organisms, from muscle contraction to protein synthesis and nerve impulse transmission. Understanding ATP's structure is key to understanding its function. This comprehensive guide will delve into the three integral parts of an ATP molecule, exploring their individual roles and the synergistic interplay that makes ATP the ultimate energy currency of life.
The Tripartite Structure: Adenine, Ribose, and Phosphate Groups
ATP is a nucleotide, a fundamental building block of nucleic acids like DNA and RNA. However, its function extends far beyond its role in genetic material. The ATP molecule's unique structure, comprised of three distinct components, is precisely what enables its remarkable energy-carrying capacity:
-
Adenine: A nitrogenous base, adenine forms one of the fundamental building blocks of DNA and RNA. Its specific planar structure, characterized by a purine ring system with two nitrogen-containing rings, contributes significantly to ATP's stability and ability to interact with other molecules within the cell. The adenine base is crucial for recognizing and interacting with enzymes involved in ATP synthesis and hydrolysis.
-
Ribose: A five-carbon sugar (pentose), ribose provides the structural backbone of the ATP molecule. Its cyclic structure acts as a scaffold, connecting the adenine base to the chain of phosphate groups. The hydroxyl (-OH) groups on the ribose sugar play a vital role in ATP's interaction with water molecules and enzymes, influencing its solubility and reactivity within the cellular environment. The specific arrangement of these hydroxyl groups distinguishes ribose from deoxyribose found in DNA, highlighting the unique nature of ATP as a distinct energy molecule.
-
Phosphate Groups: This is the most crucial part regarding ATP’s energy storage and release. Three phosphate groups (triphosphate) are linked to the ribose sugar in a high-energy phosphoanhydride bond. These bonds are characterized by a significant amount of potential energy, due to the strong electrostatic repulsion between the negatively charged phosphate groups. This repulsion is the key to ATP’s energy storage capability. It's the breaking of these high-energy phosphate bonds that releases the energy utilized for cellular processes. The hydrolysis of ATP, specifically the removal of the terminal phosphate group to form adenosine diphosphate (ADP), releases a significant amount of energy, driving various metabolic reactions.
The High-Energy Phosphate Bonds: The Secret to ATP's Power
The high-energy phosphoanhydride bonds connecting the phosphate groups are the crux of ATP's energy-carrying capacity. These bonds are not simply strong covalent bonds; their high-energy nature stems from several factors:
-
Electrostatic Repulsion: The negatively charged phosphate groups strongly repel each other. This repulsion creates an unstable configuration, storing significant potential energy. The closer these negatively charged groups are forced together, the higher the stored potential energy.
-
Resonance Stabilization: The phosphate groups exhibit resonance stabilization in their free form (inorganic phosphate or Pi). When they are bound to the ATP molecule, this resonance stabilization is lost. The difference in resonance stability between the bound and free forms contributes to the high-energy nature of the phosphoanhydride bonds.
-
Hydration: The free phosphate groups are better hydrated than those within the ATP molecule. The increased hydration of the free phosphate groups contributes to the overall energy released upon hydrolysis.
The energy released upon hydrolysis of ATP is not directly used to fuel cellular processes. Instead, it is coupled to other reactions, making thermodynamically unfavorable reactions become favorable. This coupling mechanism is a crucial aspect of cellular metabolism.
ATP Synthesis: The Processes That Generate the Energy Currency
The creation of ATP, a process called ATP synthesis, is critical for maintaining cellular energy levels. Two primary mechanisms contribute to ATP synthesis:
1. Oxidative Phosphorylation:
Oxidative phosphorylation is the primary method of ATP synthesis in aerobic organisms. This process occurs within the mitochondria, the powerhouse of the cell, and involves the electron transport chain and chemiosmosis. During this process, electrons are passed down an electron transport chain, generating a proton gradient across the inner mitochondrial membrane. This gradient drives ATP synthase, an enzyme that uses the energy stored in the proton gradient to phosphorylate ADP to ATP. The process involves a series of redox reactions, where electrons are passed from one molecule to another, releasing energy that is ultimately harnessed to produce ATP. This is a highly efficient process, producing the majority of ATP required by the cell.
2. Substrate-Level Phosphorylation:
Substrate-level phosphorylation is a less efficient but crucial mechanism of ATP synthesis. Unlike oxidative phosphorylation, it doesn't involve an electron transport chain or proton gradient. Instead, it directly transfers a phosphate group from a high-energy phosphorylated substrate to ADP, forming ATP. This process occurs during glycolysis and the citric acid cycle, producing a smaller but essential amount of ATP. This mechanism is particularly important during anaerobic conditions where oxidative phosphorylation is not possible.
ATP Hydrolysis: The Energy Release Mechanism
The release of energy from ATP is achieved through ATP hydrolysis, a reaction where water molecules break the phosphoanhydride bond between the terminal phosphate group and the rest of the molecule. This reaction produces ADP (adenosine diphosphate) and inorganic phosphate (Pi). The energy released in this reaction is not released as heat but rather is used to drive other reactions. This coupling is essential because it allows cells to perform work, such as muscle contraction or active transport across membranes.
The hydrolysis reaction is catalyzed by various enzymes, each specific to a particular cellular process. This specificity allows for the precise regulation of energy usage within the cell. The released phosphate group is often transferred to another molecule, activating it or changing its conformation, facilitating the biological process. This transfer often involves the formation of a high-energy phosphorylated intermediate, which then undergoes further reactions to carry out the desired cellular work.
The Role of ATP in Cellular Processes: A Universe of Functions
ATP's role in cellular processes is vast and pervasive. It's the fundamental energy source for a multitude of vital functions:
-
Muscle Contraction: ATP provides the energy for the myosin heads to bind to and pull on actin filaments, causing muscle contraction. The hydrolysis of ATP powers the conformational changes in the myosin molecule, resulting in the movement of the filaments and subsequent muscle shortening.
-
Active Transport: ATP fuels the action of membrane pumps that move ions and molecules against their concentration gradients. This is crucial for maintaining cellular homeostasis, for example, sodium-potassium pump that maintains the cell’s resting potential.
-
Protein Synthesis: ATP is necessary for the synthesis of proteins from amino acids. Energy is required for the activation of amino acids, formation of peptide bonds, and the translocation of mRNA and tRNA during translation.
-
Nerve Impulse Transmission: The transmission of nerve impulses requires ATP for the generation and maintenance of membrane potentials, the release of neurotransmitters, and the re-uptake of neurotransmitters.
-
DNA Replication and Repair: ATP provides the energy for the processes of DNA replication and repair, including the unwinding of the DNA double helix, the synthesis of new DNA strands, and the proofreading and correction of errors.
ATP: A Dynamic Equilibrium and its Importance for Life
The concentration of ATP in a cell is carefully regulated. The equilibrium between ATP, ADP, and Pi is a constant dynamic process, reflecting the cell's overall energy status. When the energy demands of the cell increase, the ATP concentration decreases, stimulating ATP synthesis through oxidative phosphorylation and substrate-level phosphorylation. Conversely, when the energy demands decrease, the rate of ATP synthesis slows down. This intricate balance ensures that the cell maintains sufficient ATP levels to meet its energy demands, preventing energy crises that would disrupt cellular function.
Conclusion: ATP – The Master Molecule of Life
ATP, with its three integral parts – adenine, ribose, and phosphate groups – stands as a testament to the elegance and efficiency of biological systems. Its unique structure, characterized by high-energy phosphate bonds, allows it to store and release energy in a controlled manner, fueling the vast array of cellular processes essential for life. Understanding the intricacies of ATP's structure and function is fundamental to grasping the fundamental principles of biochemistry and cellular biology. Its role extends beyond merely providing energy; it serves as a central hub in metabolic regulation, allowing cells to respond dynamically to varying energy demands, ultimately ensuring the survival and function of living organisms. From the simplest single-celled organisms to the most complex multicellular beings, ATP's ubiquitous presence underlines its essential and irreplaceable role in the intricate tapestry of life.
Latest Posts
Latest Posts
-
What Is The Formula Of Lithium Sulfide
Apr 10, 2025
-
Where In The Periodic Table Are Metals Found
Apr 10, 2025
-
Given The Circumference Find The Diameter
Apr 10, 2025
-
75 Of What Number Is 66
Apr 10, 2025
-
How Are Solids And Liquids Similar
Apr 10, 2025
Related Post
Thank you for visiting our website which covers about Three Parts Of An Atp Molecule . We hope the information provided has been useful to you. Feel free to contact us if you have any questions or need further assistance. See you next time and don't miss to bookmark.