The Vsepr Theory Is Used To
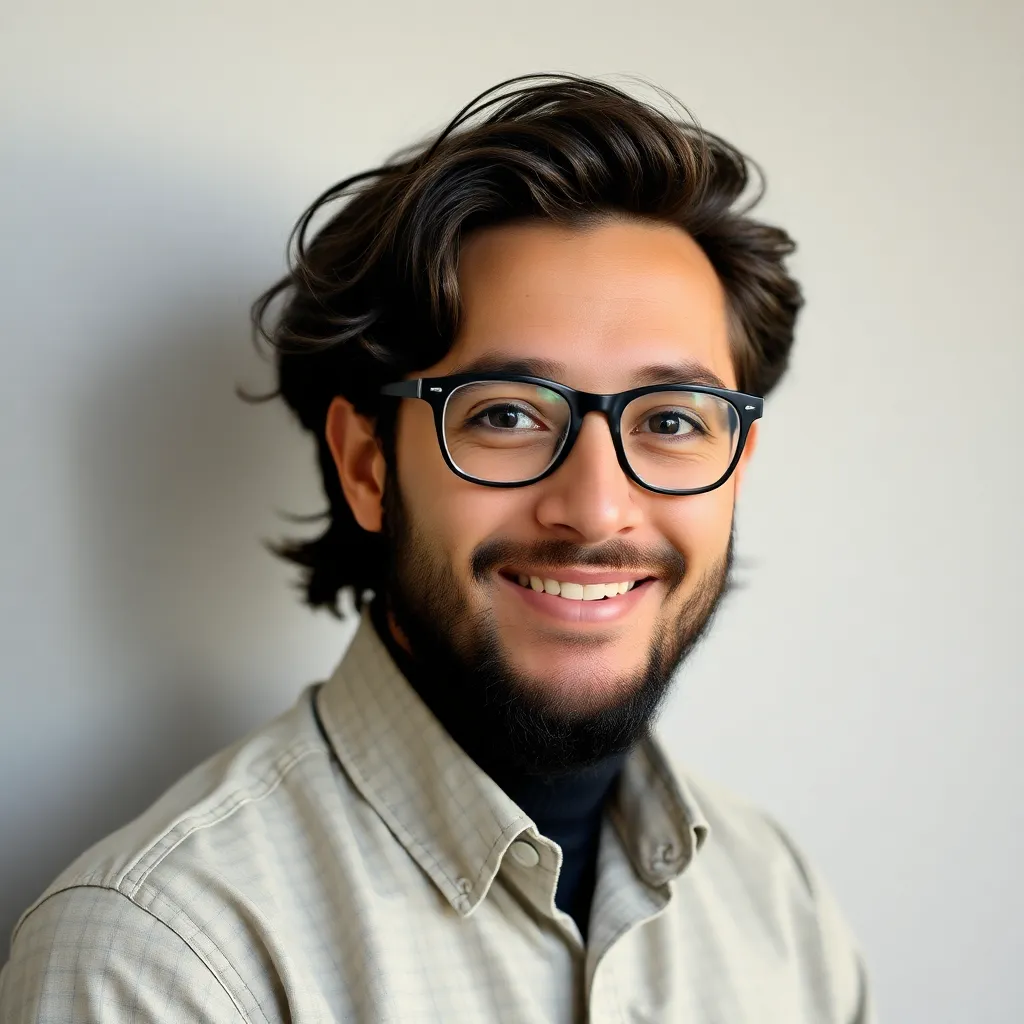
listenit
May 10, 2025 · 6 min read
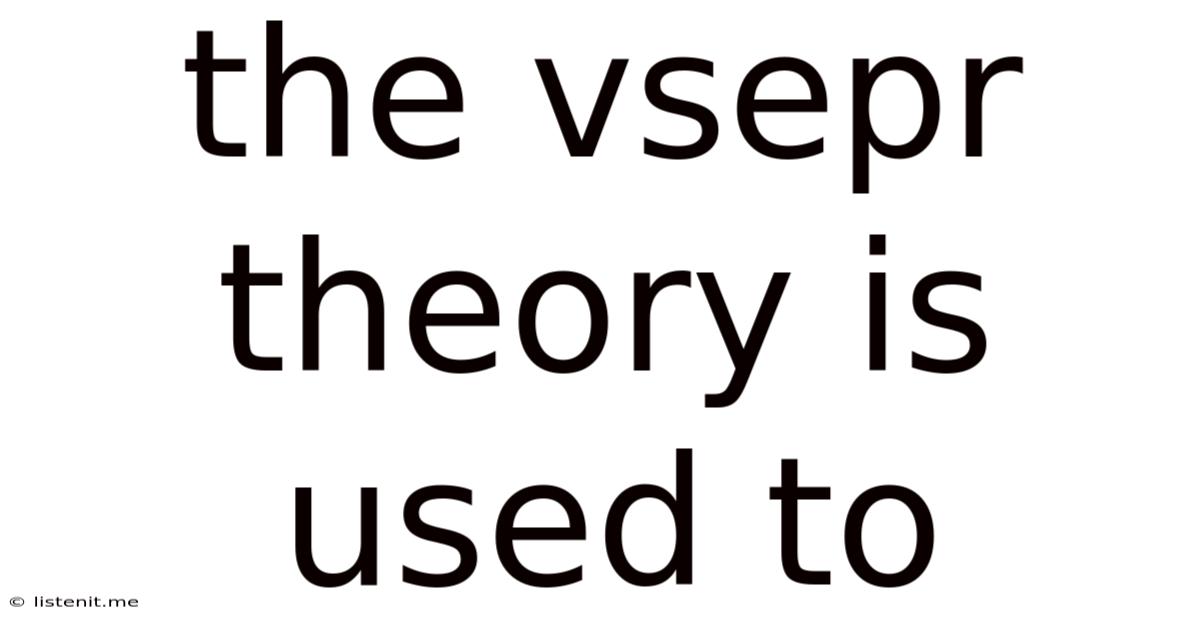
Table of Contents
The VSEPR Theory: Predicting Molecular Shapes and Understanding Reactivity
The Valence Shell Electron Pair Repulsion (VSEPR) theory is a cornerstone of chemistry, providing a simple yet powerful model for predicting the three-dimensional shapes of molecules. Understanding VSEPR is crucial for grasping the properties and reactivity of countless compounds. This comprehensive guide delves into the theory's core principles, explores its applications, and highlights its limitations.
The Fundamentals of VSEPR Theory
At its heart, VSEPR theory posits that the shape of a molecule is determined by the repulsion between electron pairs in the valence shell of the central atom. These electron pairs, whether bonding (shared between atoms) or non-bonding (lone pairs), arrange themselves as far apart as possible to minimize electrostatic repulsion. This arrangement dictates the overall geometry of the molecule.
Key Concepts and Terminology:
- Electron Domains: This term encompasses both bonding pairs and lone pairs of electrons around the central atom. Each domain occupies a region of space.
- Bonding Pairs: These are pairs of electrons shared between the central atom and a surrounding atom, forming a covalent bond.
- Lone Pairs (Non-bonding Pairs): These are pairs of electrons that are not involved in bonding and remain localized on the central atom. They exert a stronger repulsive force than bonding pairs.
- Steric Number: This is the total number of electron domains (bonding pairs + lone pairs) around the central atom. It's a crucial factor in determining the molecular geometry.
Predicting Molecular Shapes using VSEPR
The steric number is the key to predicting the shape. Different steric numbers lead to different arrangements of electron domains, resulting in distinct molecular geometries. Here's a breakdown of common geometries:
Steric Number 2: Linear Geometry
When the steric number is 2 (two electron domains), the electron pairs arrange themselves linearly, 180° apart. Examples include BeCl₂ and CO₂. Linear molecules are characterized by a straight line arrangement of atoms.
Steric Number 3: Trigonal Planar Geometry
With three electron domains (steric number 3), the optimal arrangement is trigonal planar, with bond angles of approximately 120°. Examples include BF₃ and SO₃. Trigonal planar molecules have atoms arranged in a flat triangle.
Steric Number 4: Tetrahedral Geometry
A steric number of 4 leads to a tetrahedral arrangement, with bond angles of approximately 109.5°. This is a very common geometry, exemplified by methane (CH₄) and ammonium (NH₄⁺). Tetrahedral molecules have a three-dimensional arrangement with four atoms surrounding the central atom in the shape of a tetrahedron.
Variations within Tetrahedral Geometry:
The presence of lone pairs alters the molecular geometry even if the electron domain geometry remains tetrahedral. For instance:
- Ammonia (NH₃): Three bonding pairs and one lone pair result in a trigonal pyramidal molecular geometry. The lone pair occupies more space than a bonding pair, compressing the bond angles slightly below 109.5°.
- Water (H₂O): Two bonding pairs and two lone pairs result in a bent or angular molecular geometry. The repulsion from two lone pairs significantly compresses the bond angle to approximately 104.5°.
Steric Number 5: Trigonal Bipyramidal Geometry
Five electron domains arrange themselves in a trigonal bipyramidal geometry. This arrangement consists of three equatorial positions (120° apart) and two axial positions (180° apart). Examples include PCl₅. Variations arise depending on whether the lone pairs occupy axial or equatorial positions, impacting bond angles and overall shape.
Steric Number 6: Octahedral Geometry
A steric number of 6 results in an octahedral geometry, with bond angles of 90° between adjacent atoms. Examples include SF₆. Similar to the trigonal bipyramidal case, the positioning of lone pairs influences the final molecular geometry.
Beyond Basic Shapes: More Complex Molecules
VSEPR theory can be applied to more complex molecules with multiple central atoms or branched structures. Predicting the shapes of these molecules requires a step-by-step analysis of each central atom and its surrounding electron domains.
Applying VSEPR to Larger Molecules: A Step-by-Step Approach
- Draw the Lewis structure: This step is crucial to determine the number of bonding and non-bonding electron pairs around each central atom.
- Determine the steric number for each central atom: Add the number of bonding and lone pairs for each central atom.
- Predict the electron domain geometry for each central atom: Use the steric number to determine the basic geometry (linear, trigonal planar, tetrahedral, etc.).
- Consider the influence of lone pairs: If lone pairs are present, adjust the basic geometry to account for the stronger repulsive forces exerted by the lone pairs. This will modify the bond angles and the overall molecular shape.
- Combine the geometries of individual central atoms: For larger molecules with multiple central atoms, combine the predicted geometries for each atom to obtain the overall three-dimensional shape of the molecule.
Applications of VSEPR Theory
VSEPR theory is not merely an academic exercise; it has numerous practical applications in various fields:
- Predicting Molecular Polarity: The molecular geometry, as predicted by VSEPR, is essential in determining whether a molecule is polar or nonpolar. Polarity plays a significant role in determining the physical and chemical properties of substances, such as boiling points, solubility, and reactivity.
- Understanding Reactivity: The shape of a molecule directly influences its reactivity. Steric hindrance, the spatial interference caused by the bulkiness of atoms or groups, can affect the accessibility of reaction sites.
- Spectroscopy: The predicted molecular geometries are consistent with experimental data from various spectroscopic techniques such as infrared (IR) and nuclear magnetic resonance (NMR) spectroscopy. This strengthens the theory's predictive power.
- Drug Design: In pharmaceutical chemistry, understanding molecular shapes is crucial for designing drugs that can effectively interact with specific target molecules in the body. VSEPR theory aids in predicting the shapes of drug candidates, helping researchers to optimize their design.
- Materials Science: The design of new materials with specific properties often relies on predicting the structures of molecules and their interactions. VSEPR theory contributes to this understanding.
Limitations of VSEPR Theory
While VSEPR theory is remarkably effective, it does have limitations:
- It's a simplified model: VSEPR does not explicitly consider the quantum mechanical nature of electron interactions. It provides a qualitative, rather than quantitative, understanding of molecular shapes.
- It struggles with certain molecules: Some molecules, particularly those with transition metals or multiple bonds, do not always conform perfectly to VSEPR predictions. More sophisticated computational methods are often needed in such cases.
- It doesn't predict bond lengths or bond angles precisely: While it predicts the overall geometry, it does not provide precise bond lengths and angles, which may be necessary for detailed analysis.
- It does not account for dynamic effects: Molecules may exhibit different conformations and dynamics that are not captured by the static model proposed by VSEPR.
Conclusion
The VSEPR theory remains a remarkably valuable tool for understanding and predicting the shapes of molecules. Its simplicity and predictive power make it an indispensable concept in chemistry education and research. While it has limitations and is not universally applicable, its contributions to our understanding of molecular structure and reactivity are undeniable. Combining VSEPR with more advanced computational methods provides a comprehensive approach to exploring the fascinating world of molecular shapes and their consequences. Understanding VSEPR is a crucial step towards mastering the intricacies of chemical bonding and reactivity.
Latest Posts
Latest Posts
-
How Do Convection Currents Cause Wind
May 10, 2025
-
Greatest Common Factor Of 36 And 28
May 10, 2025
-
What Is 25 As A Fraction In Simplest Form
May 10, 2025
-
How Many Neutrons Are In An Atom Of Carbon 14
May 10, 2025
-
What Is The Electron Configuration Of Se
May 10, 2025
Related Post
Thank you for visiting our website which covers about The Vsepr Theory Is Used To . We hope the information provided has been useful to you. Feel free to contact us if you have any questions or need further assistance. See you next time and don't miss to bookmark.