The Tertiary Structure Of A Polypeptide Refers To
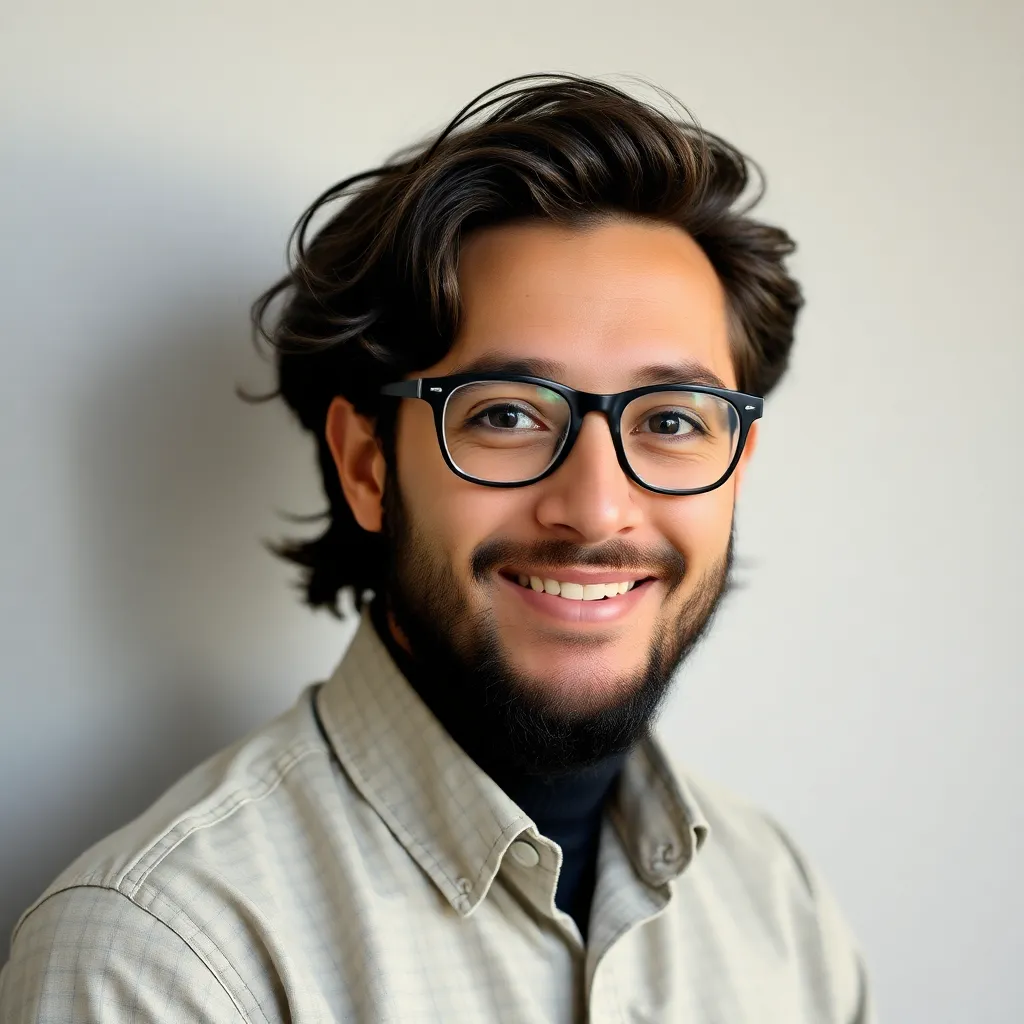
listenit
May 27, 2025 · 7 min read
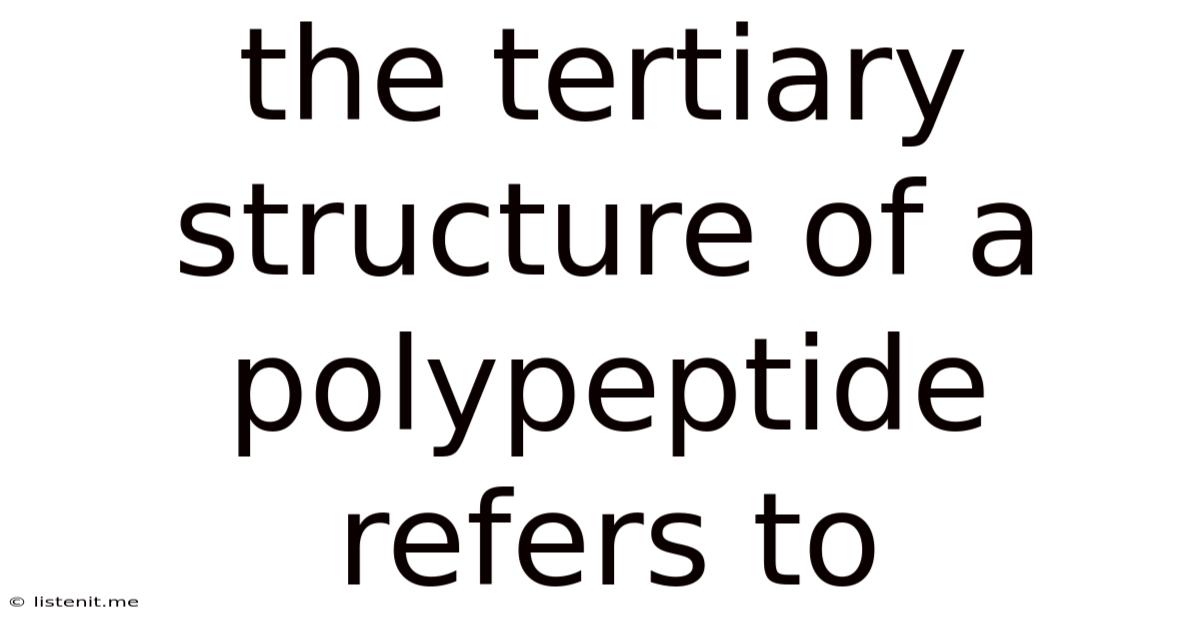
Table of Contents
The Tertiary Structure of a Polypeptide: A Deep Dive into Protein Folding
The tertiary structure of a polypeptide refers to the three-dimensional arrangement of its amino acid residues in space. This intricate folding pattern isn't random; it's dictated by a complex interplay of forces, ultimately determining the protein's function. Understanding tertiary structure is crucial in biochemistry, as it underpins the activity of enzymes, antibodies, receptors, and countless other proteins essential for life. This article will explore the intricacies of tertiary structure, including the forces driving folding, common structural motifs, and the impact of misfolding.
Forces Shaping the Tertiary Structure
The folding of a polypeptide chain into its unique tertiary structure isn't a passive process. Instead, it's driven by a multitude of non-covalent interactions, working in concert to achieve the most energetically favorable conformation. These forces include:
1. Hydrophobic Interactions: The Driving Force
Arguably the most significant force driving protein folding is the hydrophobic effect. Amino acid side chains can be categorized as hydrophobic (water-fearing) or hydrophilic (water-loving). During folding, hydrophobic residues cluster together in the protein's interior, minimizing their contact with the surrounding aqueous environment. This aggregation is entropically driven, as it increases the disorder of the water molecules surrounding the protein. The hydrophobic core acts as a scaffold around which the rest of the structure is built.
2. Hydrogen Bonds: Stabilizing the Structure
Hydrogen bonds, while individually weak, collectively contribute significantly to protein stability. These bonds form between the polar side chains of amino acids and/or the peptide backbone. They frequently link secondary structural elements like alpha-helices and beta-sheets together, stabilizing their relative orientations within the tertiary structure. Hydrogen bonds also play a crucial role in stabilizing interactions between different regions of the polypeptide chain that are not directly adjacent in the primary sequence.
3. Ionic Interactions (Salt Bridges): Electrostatic Attractions
Ionic interactions, or salt bridges, occur between oppositely charged amino acid side chains (e.g., a negatively charged aspartate and a positively charged lysine). These electrostatic attractions can be quite strong, contributing significantly to the stability of the protein's tertiary structure. The strength of these interactions is influenced by the dielectric constant of the surrounding environment; a lower dielectric constant (like the protein's hydrophobic core) strengthens these interactions.
4. Disulfide Bonds: Covalent Cross-links
Disulfide bonds are strong covalent bonds formed between the sulfur atoms of cysteine residues. These bonds act as cross-links, reinforcing the tertiary structure and significantly enhancing the protein's stability. They're particularly important in extracellular proteins, which are exposed to harsher conditions than intracellular proteins. The formation of disulfide bonds is an oxidative process, often catalyzed by enzymes.
5. Van der Waals Forces: Weak but Numerous
Van der Waals forces are weak, short-range attractions between atoms due to temporary fluctuations in electron distribution. While individually weak, the cumulative effect of numerous van der Waals forces throughout the protein can make a substantial contribution to overall stability. These forces are especially significant in packing the hydrophobic core of the protein, ensuring close interactions between tightly packed side chains.
Common Structural Motifs in Tertiary Structure
The tertiary structure isn't just a random jumble of amino acids; it often incorporates recurring structural motifs, patterns that are frequently observed in diverse proteins. These motifs often contribute to the protein's function or stability. Some common motifs include:
1. α-Helices and β-Sheets: Building Blocks
While alpha-helices and beta-sheets are elements of secondary structure, their relative arrangement and interactions within the tertiary structure are critical. The packing of these secondary structure elements, along with connecting loops, significantly contributes to the overall three-dimensional fold. For instance, a protein may have a core made primarily of beta-sheets surrounded by alpha-helices.
2. β-Turns and Loops: Connecting Secondary Structures
β-turns and loops are short segments of the polypeptide chain that connect alpha-helices and beta-sheets. They often contain glycine or proline residues, which are structurally flexible, allowing them to accommodate sharp turns. These turns are essential in determining the overall shape of the protein and the relative orientation of its secondary structure elements.
3. Zinc Fingers and Other Metal-Binding Domains
Many proteins incorporate metal ions into their tertiary structures, forming metal-binding domains. These domains, such as zinc fingers, use zinc ions to stabilize a specific structural motif crucial for their function, such as DNA binding. The metal ions act as structural scaffolds, coordinating the positions of specific amino acid residues.
4. Coiled-Coils: Two or More α-Helices Wrapped Around Each Other
Coiled-coils are structural motifs formed by two or more alpha-helices wrapping around each other. The helices are stabilized by hydrophobic interactions between their side chains. Coiled-coils are frequently found in structural proteins, contributing to strength and stability.
Predicting Tertiary Structure: From Sequence to Shape
Predicting the tertiary structure of a protein from its amino acid sequence remains a significant challenge in bioinformatics. While considerable progress has been made, accurately predicting the complex three-dimensional arrangement of a protein is still computationally demanding. Various approaches are used, including:
1. Homology Modeling: Leveraging Known Structures
Homology modeling involves using the known structure of a similar protein (a homologue) as a template to predict the structure of a new protein with a similar sequence. This approach relies on the principle that proteins with similar sequences often have similar structures.
2. Ab initio Methods: From Scratch Prediction
Ab initio methods attempt to predict the tertiary structure without using any template structures. These methods use sophisticated algorithms to evaluate the energy of different conformations, searching for the lowest-energy state, which represents the most stable structure. These methods are highly computationally intensive.
3. Threading Methods: Matching Sequences to Structural Databases
Threading methods attempt to fit a target protein sequence onto known protein structural folds. This approach involves comparing the sequence with a library of known protein structures and identifying the best match.
The Impact of Misfolding: Diseases and Aggregates
The precise folding of a protein is crucial for its function. Misfolding can result in a loss of function or the formation of aggregates, which can be harmful to the cell. Protein misfolding is implicated in many diseases, including:
1. Alzheimer's Disease: Amyloid Plaques
Alzheimer's disease is characterized by the accumulation of amyloid plaques, which are aggregates of misfolded amyloid-beta protein. These plaques disrupt neuronal function and contribute to the cognitive decline associated with the disease.
2. Parkinson's Disease: Lewy Bodies
Parkinson's disease is associated with the accumulation of Lewy bodies, aggregates of misfolded alpha-synuclein. These aggregates disrupt neuronal function and contribute to the motor symptoms of the disease.
3. Prion Diseases: Infectious Misfolding
Prion diseases are caused by the misfolding of prion proteins. The misfolded form can induce further misfolding of normal prion proteins, leading to a chain reaction of misfolding and the formation of amyloid fibrils, ultimately leading to neuronal damage.
4. Cystic Fibrosis: Misfolded CFTR Protein
In cystic fibrosis, the cystic fibrosis transmembrane conductance regulator (CFTR) protein is misfolded, leading to its degradation before it reaches the cell membrane. This prevents the proper functioning of chloride channels and leads to the characteristic symptoms of the disease.
Conclusion: The Significance of Tertiary Structure
The tertiary structure of a polypeptide is a testament to the intricate complexity of biological systems. The precise three-dimensional arrangement of amino acid residues, governed by a delicate balance of forces, dictates the protein's function and stability. Understanding tertiary structure is crucial for advancing our knowledge of protein function, developing new therapies for protein-misfolding diseases, and designing new proteins with specific properties. As research continues, we can expect further breakthroughs in our understanding of this fundamental aspect of life. The interplay of forces, common motifs, and the potential for misfolding all contribute to a fascinating and complex field of study within biochemistry and molecular biology. Further research into protein folding and stability will undoubtedly lead to advancements in biotechnology and medicine.
Latest Posts
Latest Posts
-
Which Of The Following Is Predominantly Made Up Of Myosin
May 28, 2025
-
Groups Of Interconnected Neurons Are Referred To As
May 28, 2025
-
Non Lactose Fermenting Gram Negative Bacteria
May 28, 2025
-
The Pneumotaxic Center Of The Pons
May 28, 2025
-
What Is Post And Core In Dentistry
May 28, 2025
Related Post
Thank you for visiting our website which covers about The Tertiary Structure Of A Polypeptide Refers To . We hope the information provided has been useful to you. Feel free to contact us if you have any questions or need further assistance. See you next time and don't miss to bookmark.