The Shape Of A Protein Is Determined By
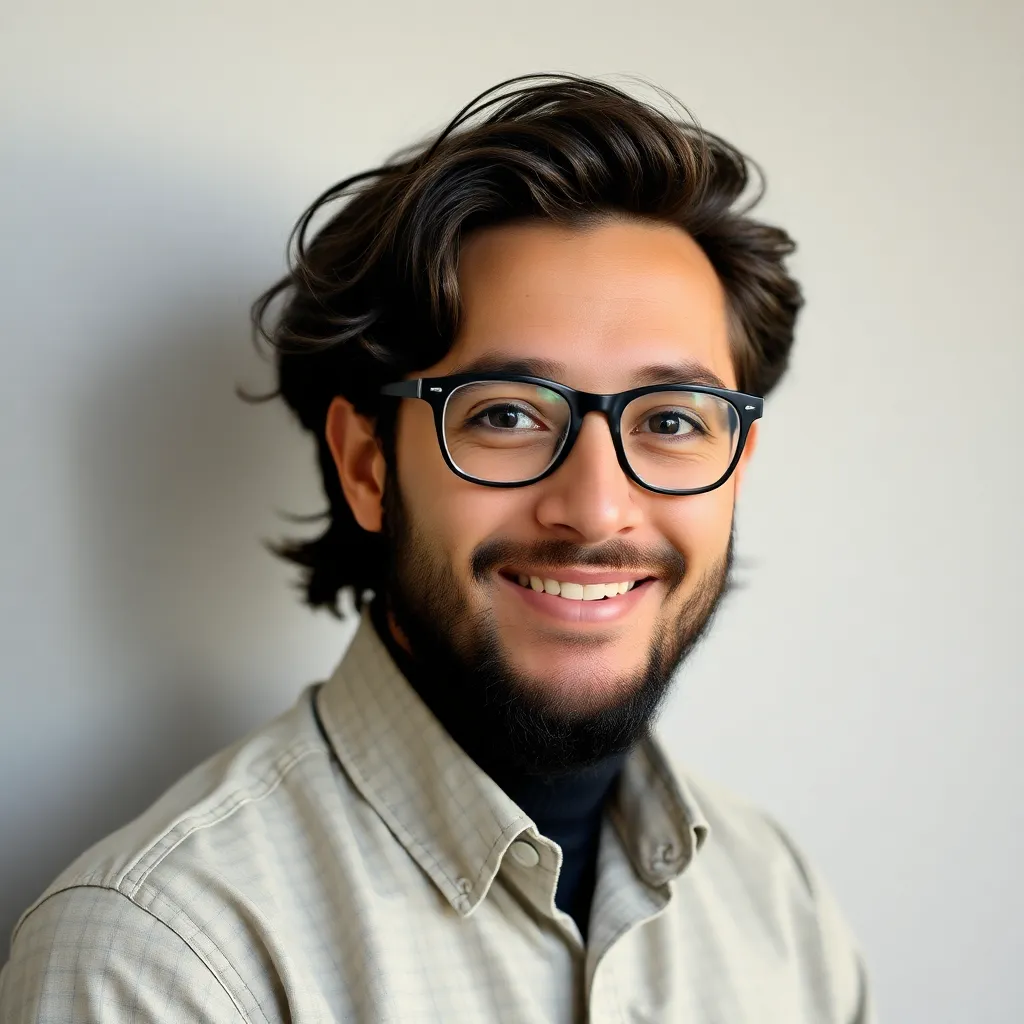
listenit
Apr 20, 2025 · 6 min read

Table of Contents
The Shape of a Protein: Determined by a Complex Dance of Forces
The shape of a protein, its three-dimensional structure, is not arbitrary. It's a meticulously crafted masterpiece, sculpted by a complex interplay of forces and dictated by its amino acid sequence. This intricate structure is absolutely crucial to its function; a slight alteration can render a protein useless or even harmful. Understanding how a protein achieves its final shape, therefore, is fundamental to comprehending biology itself. This article will delve deep into the fascinating world of protein folding, exploring the key factors that determine a protein's unique conformation.
The Primary Structure: The Blueprint of the Protein
The journey to understanding protein shape begins with its primary structure – the linear sequence of amino acids. This sequence, dictated by the genetic code, is akin to a blueprint. Each amino acid, with its unique side chain (R-group), contributes specific chemical properties to the overall polypeptide chain. The interactions between these side chains, along with the backbone of the protein itself, are the driving forces behind the protein's folding. This primary structure isn't just a random string of amino acids; the precise order is genetically encoded and crucial for the final three-dimensional structure. Mutations, even single amino acid substitutions, can drastically alter the protein's shape and function, often leading to disease. For example, sickle cell anemia results from a single amino acid change in the hemoglobin protein.
The Role of Peptide Bonds
The amino acids are linked together by peptide bonds, strong covalent bonds formed between the carboxyl group of one amino acid and the amino group of the next. These bonds are crucial for maintaining the integrity of the polypeptide chain and influence the overall conformation due to their inherent rigidity and planarity. The peptide bond's partial double-bond character restricts rotation around the bond itself, influencing the local geometry and contributing to the overall protein fold.
Secondary Structure: Local Folding Patterns
Once the primary structure is established, the polypeptide chain begins to fold into local structural motifs known as secondary structures. These are stabilized by hydrogen bonds between the backbone atoms (carbonyl oxygen and amide hydrogen) of the polypeptide chain. The most common secondary structures are:
Alpha-Helices: Spiral Structures
Alpha-helices are right-handed coiled structures stabilized by hydrogen bonds between the carbonyl oxygen of one amino acid and the amide hydrogen of the amino acid four residues down the chain. This creates a rod-like structure with a characteristic pitch and diameter. The side chains of the amino acids extend outwards from the helix. Certain amino acids are more favorable to alpha-helix formation than others due to their side chain properties. Proline, for example, is a helix breaker due to its rigid cyclic structure.
Beta-Sheets: Extended Structures
Beta-sheets are formed by extended polypeptide chains arranged side-by-side, stabilized by hydrogen bonds between carbonyl oxygen and amide hydrogen atoms on adjacent strands. These strands can run parallel or antiparallel, influencing the hydrogen bonding pattern and overall stability. Beta-sheets contribute significantly to the overall strength and rigidity of the protein structure.
Loops and Turns: Connecting the Elements
Regions that connect alpha-helices and beta-sheets are often unstructured loops and turns. These are less ordered but still crucial for connecting the more structured elements of the protein and guiding the overall folding pathway. While less structured, these regions are not random and often play crucial roles in protein function, such as binding sites for other molecules.
Tertiary Structure: The 3D Arrangement of Secondary Structures
The tertiary structure represents the overall three-dimensional arrangement of the secondary structure elements. This is where the protein acquires its unique functional shape. The tertiary structure is stabilized by a variety of interactions, including:
Non-covalent Interactions: The Driving Forces
- Hydrophobic Interactions: Amino acids with hydrophobic (water-fearing) side chains tend to cluster together in the protein's core, away from the surrounding aqueous environment. This is a major driving force in protein folding.
- Hydrogen Bonds: Hydrogen bonds between side chains contribute to the stability of the tertiary structure. These are weaker than peptide bonds but numerous and collectively significant.
- Ionic Bonds (Salt Bridges): Electrostatic interactions between oppositely charged side chains can form salt bridges, further stabilizing the protein structure.
- Van der Waals Forces: Weak, transient interactions between atoms in close proximity contribute to the overall stability of the protein's tightly packed structure.
Disulfide Bonds: Covalent Cross-Links
Disulfide bonds are covalent bonds formed between the cysteine residues (amino acids containing a sulfhydryl group). These bonds are particularly strong and are often found in proteins that need to be resistant to denaturation. They act as cross-links, reinforcing the overall tertiary structure.
Quaternary Structure: The Assembly of Multiple Subunits
Some proteins consist of multiple polypeptide chains, or subunits, assembled together to form a functional complex. This arrangement is called the quaternary structure. The interactions between the subunits are similar to those stabilizing the tertiary structure, involving a mix of non-covalent interactions and sometimes disulfide bonds. Hemoglobin, for example, is a tetramer composed of four subunits.
Factors Influencing Protein Folding
The path to the final protein shape isn't a simple, linear process. It's a dynamic, complex process influenced by a number of factors:
- Amino Acid Sequence: The primary structure, as already mentioned, dictates the folding pathway.
- Solvent Environment: The surrounding environment, particularly the presence of water, plays a crucial role in shaping the protein. Hydrophobic interactions are largely driven by the desire to minimize contact between hydrophobic residues and water.
- Temperature: High temperatures can disrupt the weak interactions that stabilize the protein structure, leading to denaturation (unfolding).
- pH: Changes in pH can alter the charges of amino acid side chains, affecting ionic interactions and overall stability.
- Molecular Chaperones: Specialized proteins called molecular chaperones assist in the folding process, preventing aggregation and ensuring proper folding. They provide a protective environment for nascent proteins and help them reach their native state.
- Post-Translational Modifications: Modifications that occur after the protein is synthesized, such as glycosylation or phosphorylation, can influence protein folding and stability.
Protein Misfolding and Disease
When proteins fail to fold correctly, it can lead to a variety of diseases. Misfolded proteins can aggregate, forming amyloid fibrils that are associated with neurodegenerative diseases like Alzheimer's and Parkinson's. Prion diseases, like Creutzfeldt-Jakob disease, are caused by misfolded prion proteins that can convert normally folded proteins into their misfolded state. Understanding the mechanisms of protein folding is therefore crucial for developing therapeutic strategies to combat these diseases.
Conclusion
The shape of a protein is a consequence of a remarkable interplay between its amino acid sequence, a variety of non-covalent interactions, covalent bonds, and the surrounding cellular environment. The process of protein folding is incredibly complex but ultimately vital for life. From the linear sequence of amino acids to the intricate three-dimensional structures they form, this journey highlights the elegance and precision of biological systems and underscores the importance of continued research in this crucial area of biology. Understanding how proteins fold is key not only to comprehending fundamental biological processes but also to developing treatments for a wide range of diseases associated with protein misfolding.
Latest Posts
Latest Posts
-
Prove Square Root 3 Is Irrational
Apr 20, 2025
-
16 Pints Is How Many Quarts
Apr 20, 2025
-
What Is 1 2 As A Percent
Apr 20, 2025
-
Square Root Of Negative 1 Divided By 8
Apr 20, 2025
-
How Are Velocity And Acceleration Related
Apr 20, 2025
Related Post
Thank you for visiting our website which covers about The Shape Of A Protein Is Determined By . We hope the information provided has been useful to you. Feel free to contact us if you have any questions or need further assistance. See you next time and don't miss to bookmark.