The Particles In A Crystalline Solid Are Arranged
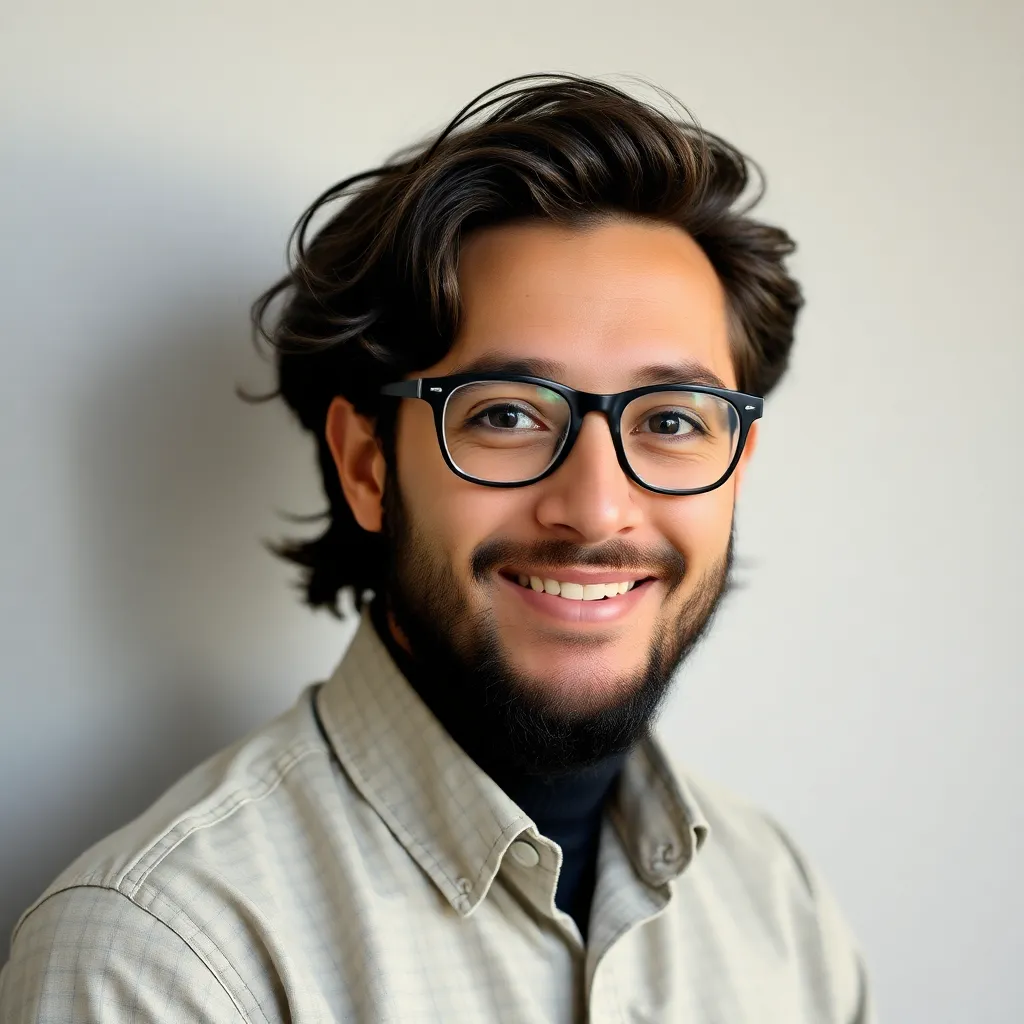
listenit
May 09, 2025 · 7 min read
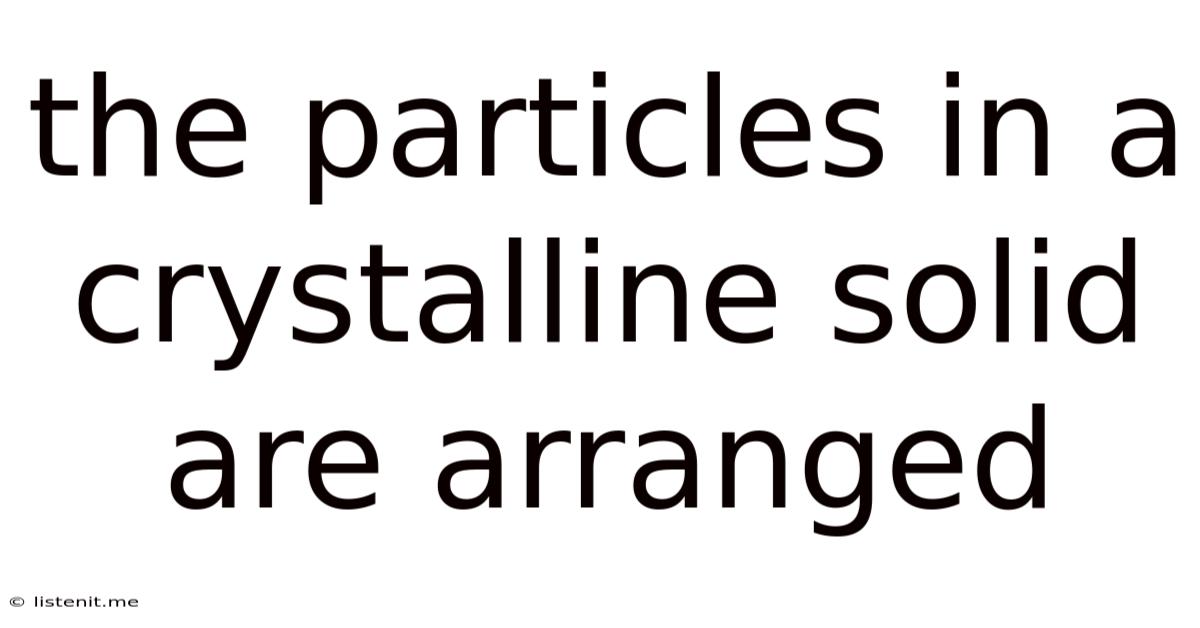
Table of Contents
The Arrangement of Particles in a Crystalline Solid: A Deep Dive
Crystalline solids are ubiquitous in our daily lives, from the silicon in our computers to the salt in our food. Their unique properties, whether it's the hardness of a diamond or the conductivity of a metal, stem directly from the highly ordered arrangement of their constituent particles – atoms, ions, or molecules. This article delves deep into the fascinating world of crystal structures, exploring the different types of arrangements, the forces holding them together, and the consequences of this ordered arrangement on macroscopic properties.
What Defines a Crystalline Solid?
Before diving into the specifics of particle arrangement, let's establish what constitutes a crystalline solid. Unlike amorphous solids (like glass) which lack long-range order, crystalline solids exhibit a highly ordered, repeating three-dimensional arrangement of their constituent particles. This repeating pattern, called a unit cell, is the fundamental building block of the crystal lattice. The unit cell repeats itself in all three spatial dimensions, extending infinitely to form the macroscopic crystal.
This long-range order is the defining characteristic of crystalline solids and directly influences their properties. This regular arrangement dictates how the particles interact with each other, affecting factors such as:
- Mechanical properties: Hardness, brittleness, ductility, and elasticity are strongly influenced by the crystal structure.
- Optical properties: The arrangement of atoms influences how light interacts with the material, leading to different colors and refractive indices.
- Electrical properties: Conductivity, whether metallic or insulating, depends heavily on the electronic structure, which is in turn determined by the atomic arrangement.
- Thermal properties: Thermal conductivity and melting point are linked to the strength of interparticle forces and the crystal structure.
Types of Crystal Structures and Unit Cells
There are several common types of crystal structures, categorized by the arrangement of their unit cells. These structures are often described using lattice parameters (the lengths of the unit cell edges) and angles between the edges. The most common Bravais lattices (fundamental unit cells) include:
1. Cubic Structures:
Cubic structures are characterized by unit cells with all edges of equal length and all angles equal to 90 degrees. There are three main types:
- Simple Cubic (SC): The simplest structure, with atoms only at the corners of the cube. It's relatively rare due to its low packing efficiency.
- Body-Centered Cubic (BCC): Atoms are located at the corners and the center of the cube. Metals like iron and tungsten exhibit this structure. BCC structures exhibit higher packing efficiency than SC.
- Face-Centered Cubic (FCC): Atoms are located at the corners and the center of each face of the cube. Metals such as aluminum, copper, and gold adopt this structure. FCC structures have the highest packing efficiency among the cubic structures.
2. Tetragonal Structures:
Similar to cubic structures but with one edge of different length. This variation in edge length leads to anisotropic properties – properties that vary depending on the direction.
3. Orthorhombic Structures:
All three edges are of different lengths, but all angles remain 90 degrees.
4. Monoclinic Structures:
Two edges of different lengths, with one angle not equal to 90 degrees.
5. Triclinic Structures:
All three edges are of different lengths, and all three angles are different from 90 degrees. This is the least symmetrical crystal system.
6. Hexagonal Structures:
These structures are characterized by a hexagonal unit cell, exhibiting unique symmetry and properties. Metals like zinc and magnesium often crystallize in this structure.
Forces Holding the Particles Together
The specific arrangement of particles in a crystal is governed by the nature of the interparticle forces. These forces dictate the stability and properties of the crystal. Different types of solids have different dominant forces:
-
Ionic Solids: These solids are held together by strong electrostatic forces between oppositely charged ions. The arrangement is dictated by the need to maximize attractive forces and minimize repulsive forces. Examples include NaCl (sodium chloride) and MgO (magnesium oxide). The crystal structure is often determined by the ionic radii and charges of the constituent ions.
-
Covalent Solids: In covalent solids, atoms are held together by covalent bonds, sharing electrons to achieve stable electron configurations. The structure is determined by the number and arrangement of covalent bonds. Diamond, a network covalent solid, is a prime example, with each carbon atom forming strong covalent bonds with four neighboring atoms in a tetrahedral arrangement. This leads to its exceptional hardness.
-
Metallic Solids: Metallic bonding involves the delocalization of valence electrons, creating a "sea" of electrons surrounding positively charged metal ions. This "sea" of electrons provides strong bonding, leading to high electrical and thermal conductivity. The specific arrangement depends on factors like atomic size and electronic structure. Many metals adopt either BCC, FCC, or HCP (Hexagonal Close-Packed) structures.
-
Molecular Solids: In molecular solids, individual molecules are held together by relatively weak intermolecular forces like van der Waals forces, hydrogen bonds, or dipole-dipole interactions. The structure is largely determined by the shape and size of the molecules and the nature of these weak forces. Examples include ice (H₂O) and solid CO₂ (dry ice). The weaker forces lead to lower melting points and greater softness compared to ionic, covalent, or metallic solids.
Consequences of Ordered Arrangement: Macroscopic Properties
The ordered arrangement of particles in crystalline solids directly translates to their macroscopic properties. Several examples illustrate this connection:
-
Anisotropy: Crystals often exhibit anisotropy, meaning their properties vary with direction. This is particularly evident in crystals with lower symmetry. For example, the cleavage properties of mica, its tendency to split along specific planes, are a direct consequence of its layered crystal structure.
-
Mechanical Strength: The strength and hardness of a crystalline solid depend on the strength of the interatomic bonds and the packing efficiency of the atoms. Close-packed structures like FCC and HCP generally exhibit higher hardness than less closely packed structures like SC.
-
Electrical Conductivity: The electrical conductivity of a material is strongly influenced by the crystal structure and the nature of its bonding. Metals, with their delocalized electrons, are excellent conductors. Ionic and covalent solids, with their localized electrons, are generally insulators, although some covalent solids can exhibit semiconductivity.
-
Optical Properties: The interaction of light with a crystal depends on the arrangement of atoms and the electronic structure. This leads to phenomena like birefringence (double refraction) in some anisotropic crystals. The color of a crystal can also be influenced by its crystal structure and the presence of impurities or defects.
Defects in Crystals: Breaking the Perfect Order
Although we often discuss ideal crystals with perfect order, real crystals always contain defects – imperfections in the regular arrangement of particles. These defects can significantly influence the material's properties:
-
Point defects: These are imperfections at a single lattice point, such as vacancies (missing atoms), interstitial atoms (extra atoms squeezed into the lattice), and substitutional atoms (atoms of a different element replacing the regular atoms).
-
Line defects (dislocations): These are imperfections along a line in the crystal lattice. Edge dislocations and screw dislocations are common types. Dislocations play a crucial role in plastic deformation (permanent changes in shape) of materials.
-
Planar defects: These are two-dimensional imperfections, such as grain boundaries (boundaries between different crystal grains) and stacking faults (errors in the stacking sequence of atomic planes).
-
Volume defects: These are three-dimensional imperfections such as voids (empty spaces within the crystal) and precipitates (small particles of a different phase).
These defects, while seemingly disruptive, can have profound effects on material properties. They can, for example, increase the strength or alter the electrical conductivity. The controlled introduction of defects is often used to tailor the properties of materials for specific applications.
Conclusion: The Importance of Crystalline Order
The ordered arrangement of particles in a crystalline solid is not merely an academic curiosity. It's the fundamental basis of the material's properties, dictating its mechanical strength, electrical conductivity, optical behavior, and much more. Understanding crystal structures and the forces holding them together is crucial in materials science and engineering, enabling the development of materials with specific tailored properties for diverse applications. From the microchip in your phone to the building materials around you, the principles of crystallography are essential in shaping our modern world. Further research in this field continues to push the boundaries of materials science, leading to the discovery and development of novel materials with enhanced properties for future technologies.
Latest Posts
Latest Posts
-
What Is The Least Common Multiple Of 32 And 48
May 11, 2025
-
Cells That Are Not Dividing Remain In The
May 11, 2025
-
What Is The Measure Of Angle Q
May 11, 2025
-
Is Numerator On Top Or Bottom
May 11, 2025
-
3 8 Divided By 1 4 As A Fraction
May 11, 2025
Related Post
Thank you for visiting our website which covers about The Particles In A Crystalline Solid Are Arranged . We hope the information provided has been useful to you. Feel free to contact us if you have any questions or need further assistance. See you next time and don't miss to bookmark.