The Energy That Is Needed To Get A Reaction Started
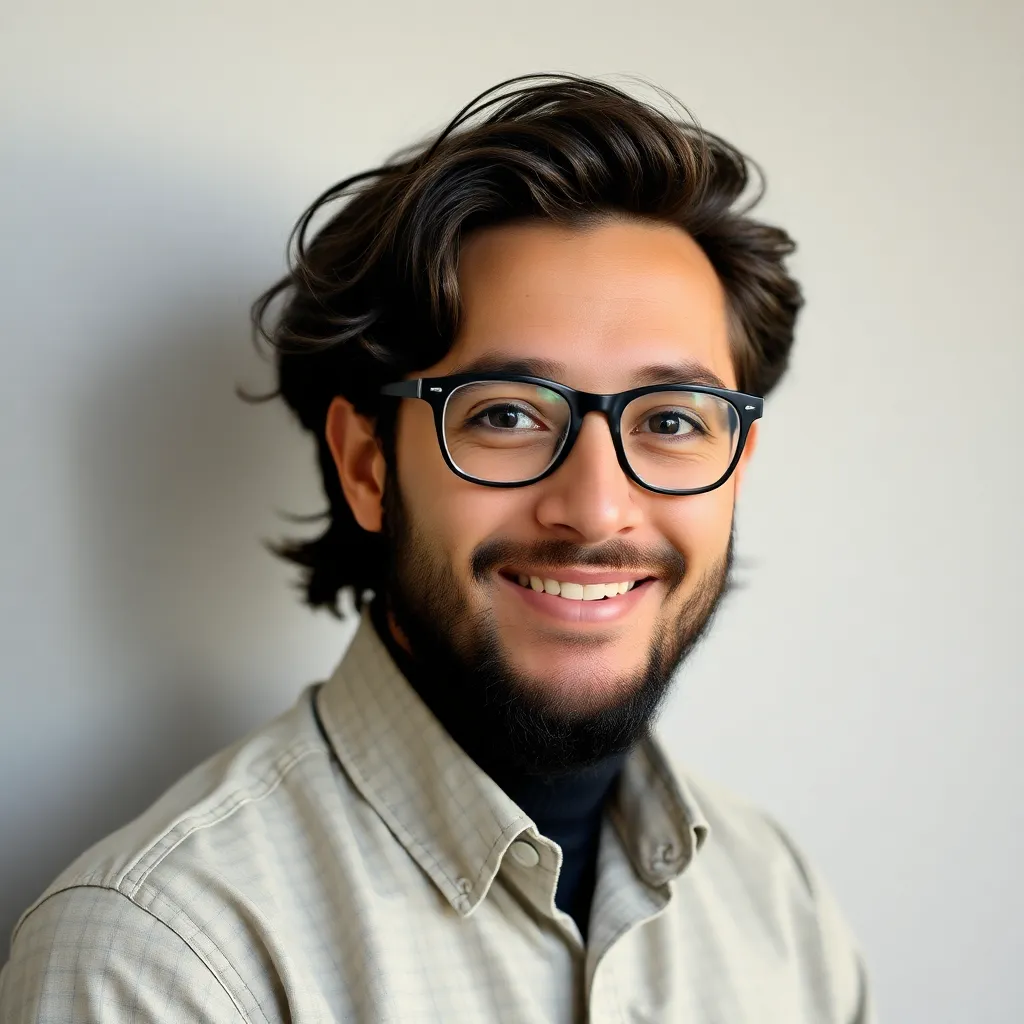
listenit
May 11, 2025 · 6 min read
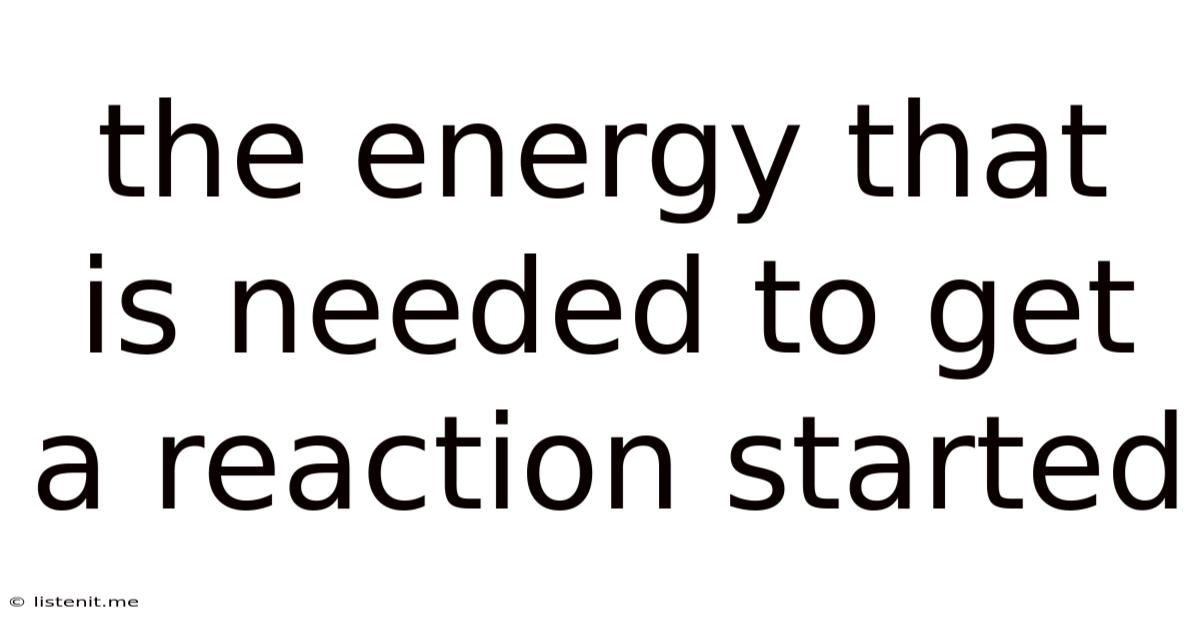
Table of Contents
The Energy Needed to Get a Reaction Started: Activation Energy Explained
Understanding chemical reactions is fundamental to comprehending the world around us. From the rusting of iron to the burning of fuel, countless processes rely on chemical transformations. However, these transformations don't spontaneously occur in every instance. There's a crucial energy barrier that needs to be overcome before a reaction can even begin – this is known as activation energy. This article delves deep into the concept of activation energy, exploring its significance, how it impacts reaction rates, and its implications across various fields.
What is Activation Energy?
Activation energy (Ea) is the minimum amount of energy required to initiate a chemical reaction. Imagine a ball resting at the top of a hill. To get it rolling down the other side, you need to give it an initial push – that push is analogous to activation energy. Molecules, similarly, require a certain amount of energy to overcome the energy barrier separating reactants from products.
This energy isn't used up in the overall reaction; instead, it's the energy needed to break existing bonds and initiate the formation of new ones. Think of it as the "investment" needed to start the reaction, with the overall reaction generating a "profit" in the form of released energy (exothermic reactions) or requiring further energy input (endothermic reactions).
The Role of Transition States
The journey from reactants to products isn't a direct path. Molecules first form a transition state, a high-energy, unstable intermediate structure. This state represents the peak of the energy barrier. Once the reactants reach this transition state, the reaction proceeds towards product formation. The activation energy is precisely the energy difference between the reactants and this transition state.
Factors Affecting Activation Energy
Several factors influence the magnitude of activation energy:
1. Nature of Reactants:
The inherent chemical structure and bonding of reactants significantly impact activation energy. Strong bonds require more energy to break than weak bonds, leading to higher activation energy. For example, reactions involving strong covalent bonds often have higher activation energies compared to those involving weaker intermolecular forces.
2. Reaction Type:
The type of reaction (e.g., substitution, addition, elimination) also influences activation energy. Certain reaction mechanisms inherently have higher energy barriers than others. For instance, reactions involving complex multi-step mechanisms often have higher activation energies than simpler one-step reactions.
3. Temperature:
Temperature plays a crucial role in overcoming the activation energy barrier. Higher temperatures provide molecules with greater kinetic energy, increasing the likelihood of successful collisions with sufficient energy to reach the transition state. This explains why reactions generally proceed faster at elevated temperatures.
4. Catalysts:
Catalysts are substances that accelerate reaction rates without being consumed in the process. They achieve this by providing an alternative reaction pathway with a lower activation energy. Catalysts essentially lower the energy barrier, making it easier for reactants to reach the transition state. This is why enzymes, biological catalysts, are essential for countless life processes. They dramatically reduce the activation energy of biochemical reactions, allowing them to proceed at biologically relevant rates.
5. Surface Area:
For reactions involving solids, the surface area of the solid reactant impacts activation energy. A larger surface area provides more sites for reactant molecules to interact, leading to a higher probability of successful collisions and thus a lower effective activation energy. This principle is utilized in various industrial processes, like catalysis, where maximizing surface area enhances reaction efficiency.
Activation Energy and Reaction Rates
The relationship between activation energy and reaction rate is inversely exponential. This is mathematically described by the Arrhenius equation:
k = Ae^(-Ea/RT)
Where:
- k is the rate constant (representing reaction speed)
- A is the pre-exponential factor (related to collision frequency)
- Ea is the activation energy
- R is the ideal gas constant
- T is the absolute temperature
This equation reveals that a higher activation energy (Ea) leads to a smaller rate constant (k), signifying a slower reaction. Conversely, a lower activation energy results in a larger rate constant and a faster reaction.
This relationship is critical in various applications, from designing efficient industrial processes to understanding the kinetics of biological systems. By manipulating factors influencing activation energy, such as temperature and catalysts, we can control reaction rates to achieve desired outcomes.
Activation Energy in Different Contexts
The concept of activation energy isn't confined to a laboratory setting; it's a ubiquitous phenomenon with far-reaching implications:
1. Industrial Chemistry:
Chemical industries heavily rely on understanding and manipulating activation energy. Optimizing reaction conditions, including temperature, pressure, and catalyst selection, is crucial for achieving high yields and efficient production. The design of industrial reactors often aims to maximize the number of successful collisions and minimize energy barriers.
2. Biochemistry:
In biological systems, enzymes act as biological catalysts, drastically reducing the activation energy of metabolic reactions. Without enzymes, many essential biochemical processes would occur too slowly to sustain life. Understanding enzyme kinetics, including their activation energies, is vital for understanding cellular function and developing pharmaceuticals.
3. Environmental Science:
Activation energy plays a crucial role in environmental processes, such as the decomposition of pollutants or the formation of greenhouse gases. Factors influencing activation energy, like temperature and sunlight, can impact the rate of these processes, which have far-reaching environmental consequences. For example, increased temperatures can accelerate the breakdown of some pollutants, while simultaneously increasing the formation rate of others.
4. Materials Science:
The synthesis and properties of materials are often dictated by activation energies involved in their formation. Controlling activation energy allows for the design of materials with specific properties, such as enhanced strength, durability, or conductivity. The understanding of activation energy is particularly important in processes such as sintering (consolidating powders into a solid mass) or crystal growth.
Measuring Activation Energy
Experimentally determining activation energy typically involves measuring the reaction rate constant at different temperatures. Plotting the natural logarithm of the rate constant (ln k) against the reciprocal of temperature (1/T) yields a straight line. The slope of this line is equal to -Ea/R, allowing for the calculation of activation energy (Ea).
This approach relies on the Arrhenius equation and its linear form:
ln k = ln A - Ea/RT
Conclusion: Activation Energy – A Cornerstone of Chemistry
Activation energy is a fundamental concept in chemistry and related fields, offering invaluable insights into the kinetics and thermodynamics of reactions. Understanding activation energy is critical for controlling reaction rates, designing efficient processes, and interpreting various natural phenomena. From industrial production to biological processes, this concept underpins a wide range of applications, showcasing its significance in our quest to unravel the complexities of the chemical world. Further research and advancements in understanding activation energy promise to yield even more significant breakthroughs in various scientific and technological domains. The ability to manipulate and control activation energy allows for the precise tailoring of chemical reactions, leading to innovations in materials science, medicine, and environmental remediation. It remains a cornerstone of chemical understanding and a crucial tool for ongoing scientific exploration.
Latest Posts
Latest Posts
-
Which Of The Following Has A Nonpolar Covalent Bond
May 12, 2025
-
What Is A Depression In A Topographic Map
May 12, 2025
-
Acceleration Of An Electron In An Electric Field
May 12, 2025
-
8 Protons 10 Neutrons 8 Electrons
May 12, 2025
-
What Is The Least Common Multiple Of 10 And 18
May 12, 2025
Related Post
Thank you for visiting our website which covers about The Energy That Is Needed To Get A Reaction Started . We hope the information provided has been useful to you. Feel free to contact us if you have any questions or need further assistance. See you next time and don't miss to bookmark.