The Electron Transport Chain Receives High-energy Electrons From
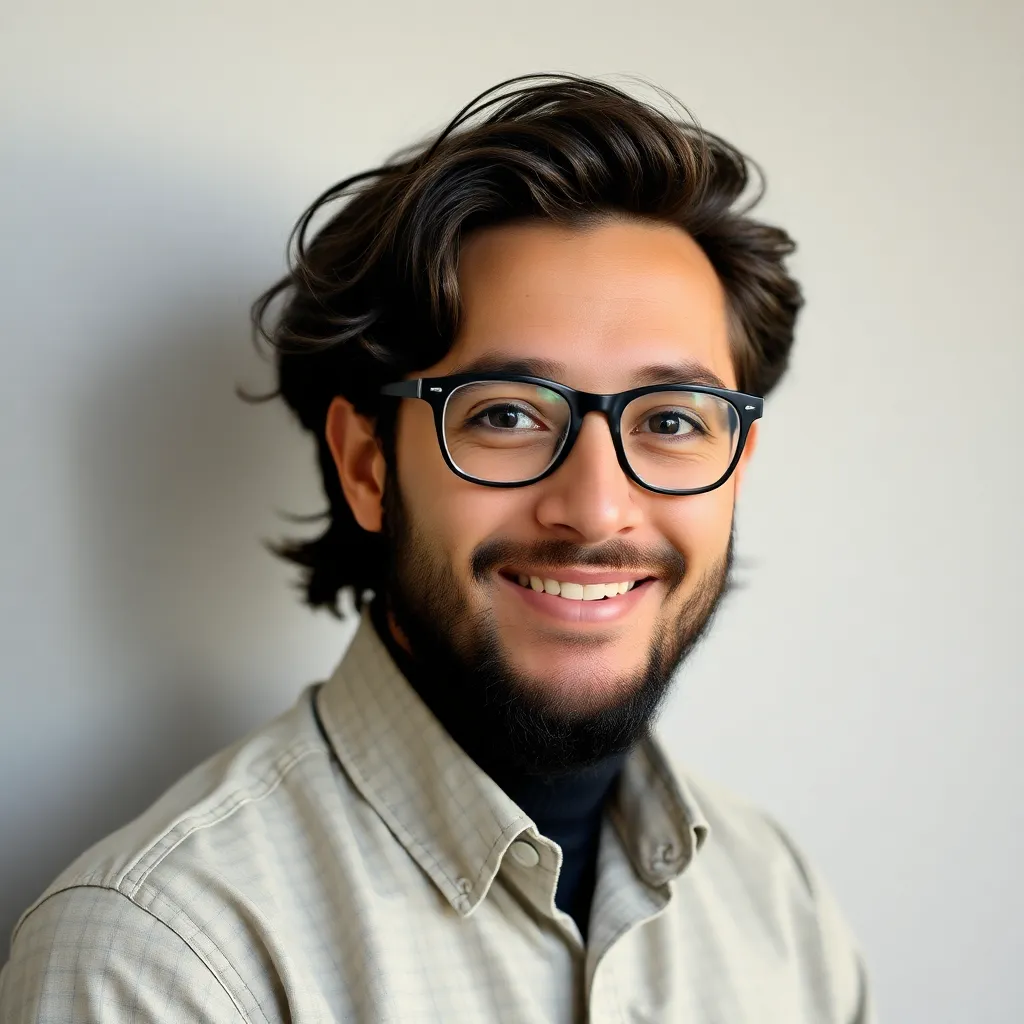
listenit
May 09, 2025 · 6 min read
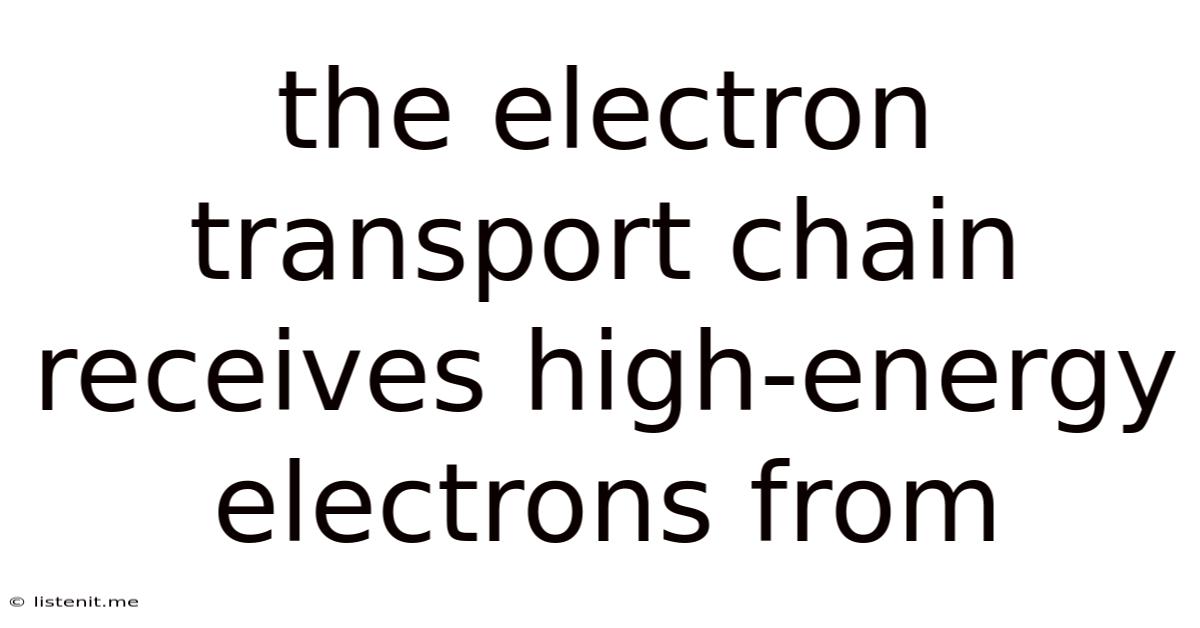
Table of Contents
The Electron Transport Chain Receives High-Energy Electrons From: A Deep Dive into Cellular Respiration
The electron transport chain (ETC), a crucial component of cellular respiration, is the powerhouse of the cell, responsible for generating the majority of ATP—the cell's energy currency. But where do these high-energy electrons that fuel the ETC originate? Understanding this is key to understanding cellular respiration itself. This article will delve into the various sources of high-energy electrons for the ETC, exploring the intricate processes involved in their generation and transfer.
NADH: The Primary Electron Donor
The primary source of high-energy electrons for the electron transport chain is NADH, a coenzyme produced during glycolysis and the citric acid cycle (also known as the Krebs cycle). NADH carries electrons in the form of a hydride ion (H⁻), which includes one proton (H⁺) and two electrons. These electrons are high-energy because they are at a relatively high reduction potential.
Glycolysis: Harvesting Energy from Glucose
Glycolysis, the initial stage of cellular respiration, takes place in the cytoplasm and breaks down glucose into two molecules of pyruvate. This process generates a small amount of ATP through substrate-level phosphorylation, but more importantly, it produces two molecules of NADH per glucose molecule. These NADH molecules then transport their high-energy electrons to the ETC.
The Citric Acid Cycle: A Central Metabolic Hub
The citric acid cycle, occurring within the mitochondrial matrix, is a cyclical series of reactions that further oxidizes the pyruvate molecules produced during glycolysis. Pyruvate is first converted into acetyl-CoA, a crucial intermediate molecule. For each acetyl-CoA molecule entering the cycle, three NADH molecules are generated. Therefore, since two pyruvate molecules are produced from one glucose molecule, a total of six NADH molecules are produced from the citric acid cycle per glucose molecule. This represents a significant contribution to the ETC's electron supply.
NADH Dehydrogenase: The Entry Point
The NADH molecules produced during glycolysis and the citric acid cycle deliver their high-energy electrons to the ETC via NADH dehydrogenase (Complex I), the first protein complex in the chain. This transfer involves the oxidation of NADH to NAD⁺ and the reduction of a molecule within Complex I, initiating the electron flow down the ETC.
FADH2: Another Key Electron Carrier
Another important electron donor to the ETC is FADH2, a coenzyme produced during the citric acid cycle. Similar to NADH, FADH2 carries high-energy electrons but at a slightly lower reduction potential than NADH. This difference in potential is crucial for the energy yield of the ETC.
FADH2's Role in the Citric Acid Cycle
The citric acid cycle generates two FADH2 molecules per glucose molecule. These FADH2 molecules transfer their electrons to a different entry point in the ETC, succinate dehydrogenase (Complex II), which is embedded within the inner mitochondrial membrane.
Bypassing Complex I: Lower Energy Yield
Because FADH2 enters the ETC at Complex II, it bypasses Complex I. This means that the electrons carried by FADH2 contribute to a smaller proton gradient across the inner mitochondrial membrane compared to those carried by NADH. Consequently, the ATP yield from FADH2 is lower than that from NADH.
Beyond NADH and FADH2: Other Minor Contributors
While NADH and FADH2 are the primary sources of electrons for the ETC, other metabolic pathways contribute to a lesser extent. These include:
- Fatty Acid Oxidation (β-oxidation): The breakdown of fatty acids generates a significant number of NADH and FADH2 molecules, contributing substantially to ATP production, especially during periods of fasting or intense exercise. These molecules then feed directly into the ETC.
- Amino Acid Oxidation: The catabolism of amino acids, the building blocks of proteins, also produces NADH and FADH2 molecules. This contributes to the overall electron supply to the ETC, although the relative contribution varies depending on the specific amino acid and metabolic conditions.
- Glycerol-3-phosphate shuttle: This shuttle system transports cytosolic NADH electrons into the mitochondria, effectively contributing to ETC activity, although the exact mechanism and ATP yield can vary across organisms and tissues.
The Electron Transport Chain: A Cascade of Redox Reactions
The ETC is a series of protein complexes embedded within the inner mitochondrial membrane. Electrons are passed down this chain through a series of redox reactions – reduction (gain of electrons) and oxidation (loss of electrons) – from a higher energy state to a lower energy state. This electron flow drives the pumping of protons (H⁺) from the mitochondrial matrix into the intermembrane space, creating a proton gradient.
The Four Complexes: A Step-by-Step Process
The four major protein complexes of the ETC (I-IV) facilitate this electron transfer:
- Complex I (NADH dehydrogenase): Receives electrons from NADH and transfers them to ubiquinone (Q), a mobile electron carrier. This process pumps protons.
- Complex II (Succinate dehydrogenase): Receives electrons from FADH2 and transfers them to ubiquinone (Q). This complex does not pump protons.
- Complex III (cytochrome bc1 complex): Receives electrons from ubiquinone (QH2) and transfers them to cytochrome c, another mobile electron carrier. This process pumps protons.
- Complex IV (cytochrome c oxidase): Receives electrons from cytochrome c and transfers them to molecular oxygen (O2), the final electron acceptor. This process pumps protons and forms water.
The Role of Mobile Electron Carriers
Ubiquinone (Q) and cytochrome c act as mobile electron carriers, shuttling electrons between the stationary complexes. Their mobility is crucial for efficient electron transport.
Chemiosmosis: Harnessing the Proton Gradient
The proton gradient generated by the ETC is the driving force behind chemiosmosis, the process by which ATP is synthesized. The protons flow back into the mitochondrial matrix through ATP synthase, an enzyme that utilizes the energy of this proton flow to phosphorylate ADP to ATP. This is oxidative phosphorylation, the major ATP-producing step of cellular respiration.
Regulation of the Electron Transport Chain
The activity of the ETC is tightly regulated to meet the cell's energy demands. Several factors influence the rate of electron flow:
- Oxygen Availability: Oxygen is the final electron acceptor; therefore, its availability is crucial for ETC function. In the absence of oxygen, the ETC shuts down, leading to a cessation of ATP production through oxidative phosphorylation.
- Substrate Availability: The availability of NADH and FADH2, the electron carriers, directly impacts the ETC's activity. Increased substrate availability leads to increased electron flow and ATP production.
- Inhibitors and Uncouplers: Certain molecules can inhibit the ETC, blocking electron flow and reducing ATP production. Others can uncouple electron transport from ATP synthesis, dissipating the proton gradient and reducing ATP yield.
Conclusion: A Complex Interplay for Cellular Energy
The electron transport chain receives high-energy electrons from a variety of sources, primarily NADH and FADH2 produced during glycolysis and the citric acid cycle. The intricate interplay of these processes, coupled with the precise regulation of the ETC, ensures efficient energy production within the cell. Understanding the origin and fate of these high-energy electrons is crucial to comprehending the fundamental processes of cellular respiration and energy metabolism. Further research into the intricacies of the ETC continues to unravel the complexities of cellular energy production, offering potential avenues for therapeutic interventions in various metabolic disorders.
Latest Posts
Latest Posts
-
0 24 As A Fraction In Simplest Form
May 11, 2025
-
How Did Matthias Schleiden Contribute To The Cell Theory
May 11, 2025
-
Atoms Of Oxygen Have A Total Of 8 Electrons
May 11, 2025
-
Solve For X Ax By C
May 11, 2025
-
Half Of 1 And 1 2 Tablespoons
May 11, 2025
Related Post
Thank you for visiting our website which covers about The Electron Transport Chain Receives High-energy Electrons From . We hope the information provided has been useful to you. Feel free to contact us if you have any questions or need further assistance. See you next time and don't miss to bookmark.