The Backbone Of A Nucleic Acid Molecule Is Made Of
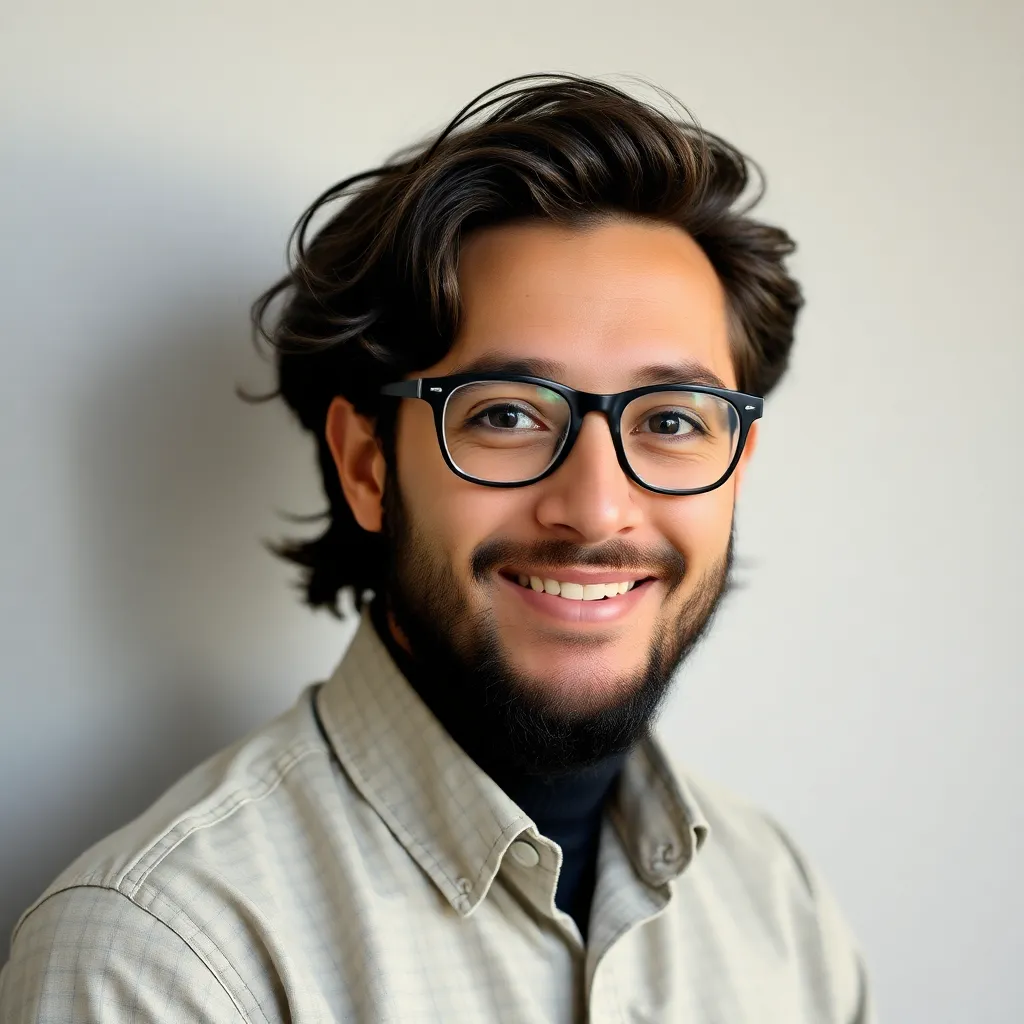
listenit
May 11, 2025 · 7 min read
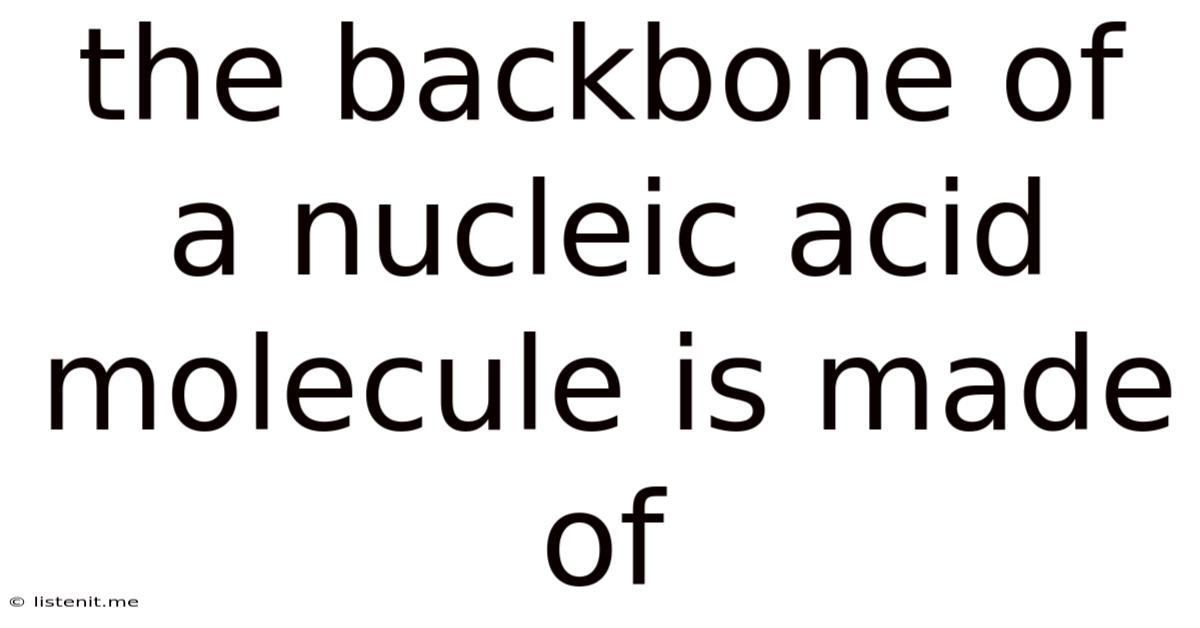
Table of Contents
The Backbone of a Nucleic Acid Molecule: A Deep Dive into Sugar-Phosphate Bonds
The backbone of a nucleic acid molecule is arguably its most fundamental structural feature, dictating its overall shape, stability, and ultimately, its function. Understanding this backbone is crucial to comprehending how DNA and RNA store and transmit genetic information, and how these processes are manipulated in various biological and technological contexts. This article will delve into the intricate details of the nucleic acid backbone, exploring its chemical composition, structural characteristics, and the implications of its unique properties.
The Building Blocks: Sugars and Phosphates
The nucleic acid backbone is a polymer composed of repeating units of sugar and phosphate groups. These units are linked together in a specific manner to form the characteristic chain.
Deoxyribose in DNA and Ribose in RNA
The sugar component is the defining difference between DNA (deoxyribonucleic acid) and RNA (ribonucleic acid). DNA employs deoxyribose, a five-carbon sugar lacking an oxygen atom on the 2' carbon. RNA, on the other hand, uses ribose, a five-carbon sugar with an oxygen atom present on the 2' carbon. This seemingly minor difference has profound implications for the overall structure and stability of the two molecules. The absence of the 2'-hydroxyl group in deoxyribose makes DNA more resistant to alkaline hydrolysis, contributing to its greater stability.
Phosphate Group: The Connecting Link
The phosphate group, a negatively charged molecule (PO₄³⁻), acts as the crucial link between the sugar units of the backbone. It connects the 3' carbon of one sugar to the 5' carbon of the next, forming a 3'-5' phosphodiester bond. This linkage is vital because it gives the backbone its directionality: a 5' end and a 3' end. This directionality is essential for many biological processes, including DNA replication and transcription. The negative charges on the phosphate groups also contribute to the overall negative charge of the nucleic acid molecule, influencing its interactions with proteins and other molecules.
The Phosphodiester Bond: The Key to the Backbone's Integrity
The phosphodiester bond is the covalent bond that joins the 3' carbon of one sugar molecule to the 5' carbon of the next sugar molecule via the phosphate group. This bond is formed through a dehydration reaction, where a water molecule is released. The strength of this bond is vital for maintaining the structural integrity of the nucleic acid molecule. It resists hydrolysis (breakdown by water), although the bond can be broken under specific conditions by enzymes like nucleases.
Formation of the Phosphodiester Bond: A Step-by-Step Look
The formation of the phosphodiester bond is a complex process involving several enzymatic steps, but the fundamental principle involves the nucleophilic attack of the 3'-hydroxyl group on the phosphate group attached to the 5'-hydroxyl group of the adjacent nucleotide. This attack displaces a hydroxyl group from the phosphate, resulting in the formation of the phosphodiester bond and the release of a water molecule. This reaction requires energy and is often driven by ATP hydrolysis.
Structural Consequences of the Backbone: Double Helix and Beyond
The sugar-phosphate backbone doesn't just hold the nucleotides together; it dictates the overall three-dimensional structure of the nucleic acid molecule. This is most clearly seen in the double helix structure of DNA.
The DNA Double Helix: A Backbone-Driven Architecture
The two strands of the DNA double helix run antiparallel to each other, meaning that one strand runs in the 5' to 3' direction, while the other runs in the 3' to 5' direction. This antiparallel arrangement is a direct consequence of the 3'-5' phosphodiester bonds in the backbone. The negatively charged phosphate groups repel each other, influencing the spatial arrangement of the two strands and contributing to the overall stability of the double helix. The hydrophobic bases are stacked in the interior, shielded from the aqueous environment by the hydrophilic sugar-phosphate backbone.
RNA's Diverse Structures: Flexibility of the Backbone
While DNA usually exists as a double helix, RNA exhibits a greater diversity of structures. The presence of the 2'-hydroxyl group in ribose makes RNA more flexible than DNA, allowing it to fold into complex secondary and tertiary structures. These structures are crucial for the various functions of RNA molecules, including mRNA, tRNA, and rRNA. The flexibility of the RNA backbone allows for the formation of hairpin loops, stem-loops, and other complex folds that are essential for RNA's function as a catalytic molecule (ribozyme) and its roles in translation and gene regulation.
Modifications to the Backbone: Expanding Nucleic Acid Functionality
The basic sugar-phosphate backbone can undergo various modifications that alter the properties and function of nucleic acids.
Methylation: Epigenetic Regulation
Methylation of the backbone, particularly of cytosine bases in DNA, is a crucial epigenetic modification. This modification does not alter the primary sequence but can alter gene expression patterns, influencing cellular differentiation and development. Methylation of the backbone can influence the interactions between DNA and proteins, influencing processes like DNA replication and repair.
Other Modifications: Impact on Stability and Function
Other modifications to the sugar-phosphate backbone include glycosylation and phosphorylation. These changes can influence the stability, interactions, and functions of nucleic acid molecules. Some modifications might enhance the stability of RNA molecules, while others might affect the binding of proteins to DNA or RNA.
The Backbone's Role in Biological Processes
The sugar-phosphate backbone is not merely a structural component; it plays an active role in various cellular processes.
DNA Replication: A Backbone-Dependent Process
The process of DNA replication hinges on the directionality of the backbone. DNA polymerase, the enzyme that synthesizes new DNA strands, can only add nucleotides to the 3' end of a growing strand. This leads to the formation of leading and lagging strands during replication. The integrity of the phosphodiester bonds is crucial for accurate and efficient replication.
Transcription: From DNA to RNA
Similarly, transcription, the process of synthesizing RNA from a DNA template, also relies on the backbone's properties. RNA polymerase, the enzyme responsible for transcription, moves along the DNA template in the 3' to 5' direction, synthesizing RNA in the 5' to 3' direction. The accuracy of transcription is dependent on the structural integrity of the DNA backbone.
RNA Processing and Translation: Backbone's Continued Influence
The RNA backbone’s structure and flexibility are crucial for RNA processing steps, such as splicing and capping, which prepare mRNA for translation. During translation, the interactions between mRNA, tRNA, and ribosomes heavily rely on the structure and properties of the RNA backbone.
Technological Applications: Harnessing the Backbone's Properties
The understanding of the nucleic acid backbone has paved the way for significant advancements in biotechnology and medicine.
PCR: Amplifying DNA
Polymerase Chain Reaction (PCR) relies on the principles of DNA replication, which in turn are intimately tied to the properties of the DNA backbone. PCR enables the exponential amplification of specific DNA sequences, a fundamental technique in molecular biology and diagnostics.
Gene Editing: CRISPR-Cas Systems
CRISPR-Cas systems, powerful gene editing tools, precisely target specific DNA sequences based on their sequence and the interactions between the target DNA and the guide RNA. These interactions depend heavily on the properties of both DNA and RNA backbones.
Nucleic Acid Therapeutics: Targeting Diseases
The understanding of nucleic acid structure, particularly the backbone, is crucial in the development of nucleic acid-based therapeutics. Antisense oligonucleotides, siRNAs, and aptamers are examples of therapeutic agents that rely on interactions with the target molecule's backbone to exert their therapeutic effects.
Conclusion: The Backbone – A Foundation for Life's Processes
The sugar-phosphate backbone of nucleic acids is far more than a simple scaffold. It is a dynamic and integral part of the molecule's function, influencing its structure, stability, interactions, and role in various biological processes. A deep understanding of its composition, structure, and modifications is crucial for deciphering the complexities of life and for advancing biotechnological applications that harness the power of nucleic acids. Further research into the nuances of the nucleic acid backbone promises to unlock even greater insights into the fundamental mechanisms of life and provide new tools for tackling challenges in medicine and biotechnology. The backbone, in its seemingly simple design, truly underpins the remarkable complexity and elegance of the genetic code.
Latest Posts
Latest Posts
-
What Is The Lcm Of 16 And 18
May 12, 2025
-
How To Find All The Zeros Of A Polynomial Function
May 12, 2025
-
What Is The Fraction Of 19
May 12, 2025
-
Which Of The Following Is A Function
May 12, 2025
-
A Wave With A Frequency Of 14 Hz
May 12, 2025
Related Post
Thank you for visiting our website which covers about The Backbone Of A Nucleic Acid Molecule Is Made Of . We hope the information provided has been useful to you. Feel free to contact us if you have any questions or need further assistance. See you next time and don't miss to bookmark.