The Ability Of An Atom To Attract Electrons
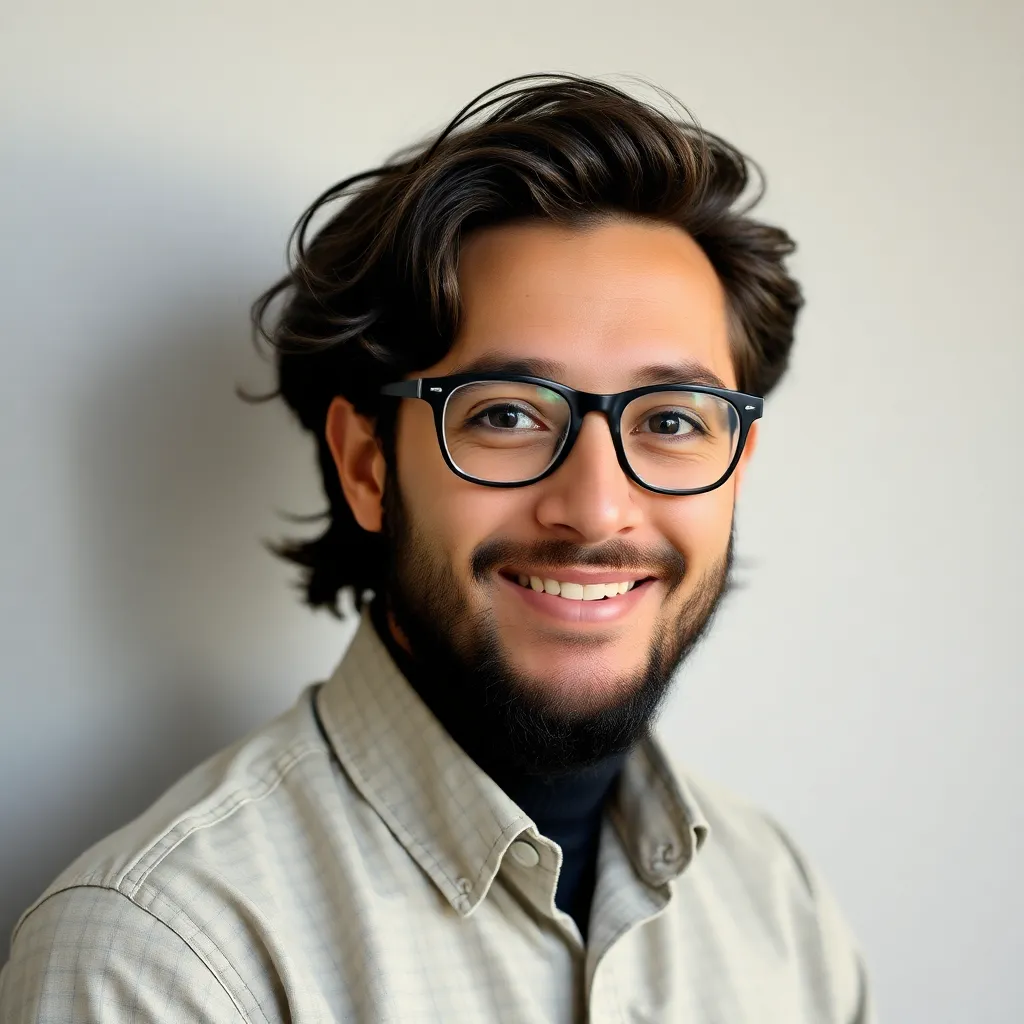
listenit
May 09, 2025 · 6 min read
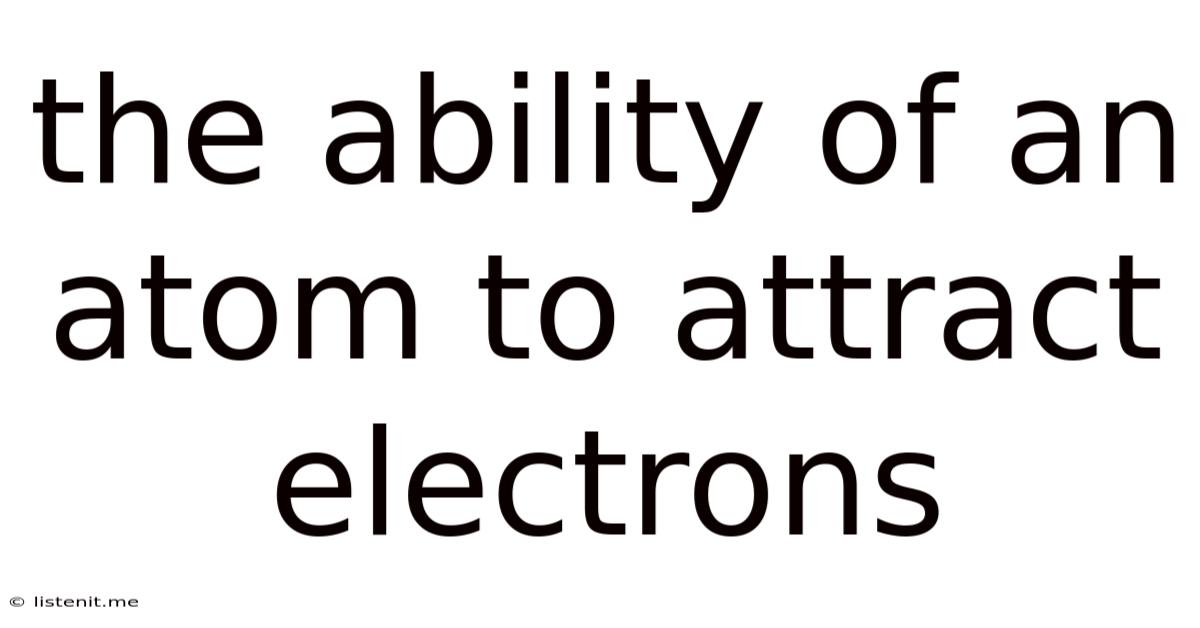
Table of Contents
The Ability of an Atom to Attract Electrons: Electronegativity and its Chemical Consequences
The ability of an atom to attract electrons is a fundamental concept in chemistry, crucial for understanding the formation of chemical bonds and the properties of molecules. This characteristic is known as electronegativity, and its influence extends far beyond the simple attraction of electrons. It dictates the polarity of bonds, the reactivity of molecules, and even the macroscopic properties of materials. This article delves deep into the nature of electronegativity, exploring its origins, trends in the periodic table, and the wide-ranging consequences it has on chemical behavior.
Understanding Electronegativity: More Than Just a Pull
Electronegativity isn't simply about the strength of an atom's pull on electrons; it's a relative measure. It compares the ability of an atom in a molecule to attract shared electrons in a chemical bond to itself. A higher electronegativity value indicates a stronger attraction for electrons. This attraction isn't a static force; it's a dynamic interplay between the atom's nucleus and the electrons involved in bonding.
The Role of Nuclear Charge and Atomic Radius
Several factors contribute to an atom's electronegativity. Nuclear charge, the positive charge of the nucleus, plays a significant role. A larger nuclear charge exerts a stronger attractive force on electrons. However, the distance between the nucleus and the valence electrons, represented by the atomic radius, also matters. A smaller atomic radius means the valence electrons are closer to the nucleus, experiencing a stronger attractive force.
These two factors are inversely related; a larger nuclear charge generally implies a smaller atomic radius (due to stronger attraction pulling the electrons closer). Therefore, atoms with a high nuclear charge and a small atomic radius tend to have high electronegativity.
Shielding Effect and Electronegativity
The shielding effect caused by inner electrons further influences electronegativity. Inner electrons partially shield the valence electrons from the full positive charge of the nucleus. This shielding reduces the effective nuclear charge experienced by the valence electrons. Atoms with more inner electrons exhibit a greater shielding effect, resulting in a lower electronegativity.
Electronegativity Trends in the Periodic Table
Electronegativity isn't a constant value; it varies systematically across the periodic table. Understanding these trends is crucial for predicting the behavior of elements and their compounds.
Electronegativity Increases Across a Period
As you move from left to right across a period (a row) in the periodic table, electronegativity generally increases. This is because the nuclear charge increases while the principal quantum number (and thus the atomic radius) remains relatively constant. The added protons increase the positive charge, pulling the valence electrons more strongly towards the nucleus.
Example: Fluorine (F) has a higher electronegativity than Lithium (Li) because it has a significantly higher nuclear charge and a similar atomic radius.
Electronegativity Decreases Down a Group
Moving down a group (a column) in the periodic table, electronegativity generally decreases. This is primarily due to the increase in atomic radius. As you add electron shells, the valence electrons are farther from the nucleus, reducing the effective nuclear charge they experience. The increased shielding effect of inner electrons also contributes to this decrease.
Example: Fluorine (F) has a higher electronegativity than Iodine (I) because the valence electrons in Iodine are significantly farther from the nucleus and more shielded.
Measuring Electronegativity: Various Scales
Several scales have been developed to quantify electronegativity. The most commonly used is the Pauling scale, developed by Linus Pauling, which assigns values ranging from approximately 0.7 (for Caesium) to 4.0 (for Fluorine). Other scales, such as the Mulliken scale and the Allred-Rochow scale, offer alternative approaches to measuring electronegativity, but the Pauling scale remains the most widely accepted.
Consequences of Electronegativity Differences: Bond Polarity and Molecular Properties
The differences in electronegativity between atoms directly influence the nature of the chemical bonds they form and the resulting properties of molecules.
Polar Covalent Bonds: Unequal Sharing
When two atoms with different electronegativities bond, they form a polar covalent bond. The more electronegative atom attracts the shared electrons more strongly, resulting in an uneven distribution of electron density. This creates a dipole moment, with one end of the bond having a partial negative charge (δ-) and the other end having a partial positive charge (δ+).
Example: In a water molecule (H₂O), oxygen is significantly more electronegative than hydrogen. This leads to polar O-H bonds, where oxygen carries a partial negative charge and hydrogen carries a partial positive charge. This polarity is crucial for water's unique properties.
Nonpolar Covalent Bonds: Equal Sharing
When two atoms with similar electronegativities bond, they form a nonpolar covalent bond. The shared electrons are distributed relatively equally between the two atoms, resulting in no significant dipole moment.
Example: In a methane molecule (CH₄), the electronegativity difference between carbon and hydrogen is small, leading to nearly nonpolar C-H bonds.
Ionic Bonds: Extreme Electronegativity Differences
When the electronegativity difference between two atoms is very large, one atom may completely transfer one or more electrons to the other, forming ions. The resulting electrostatic attraction between the oppositely charged ions constitutes an ionic bond.
Example: In sodium chloride (NaCl), chlorine is much more electronegative than sodium. Chlorine gains an electron from sodium, forming a negatively charged chloride ion (Cl⁻) and a positively charged sodium ion (Na⁺). The electrostatic attraction between these ions forms the ionic bond.
Electronegativity and Molecular Properties
The overall distribution of electron density in a molecule, determined by the electronegativities of its constituent atoms and the geometry of the molecule, significantly influences its properties.
Molecular Polarity and Intermolecular Forces
The polarity of a molecule dictates the strength of its intermolecular forces. Polar molecules exhibit dipole-dipole interactions, while nonpolar molecules experience weaker London dispersion forces. Hydrogen bonding, a particularly strong type of dipole-dipole interaction, occurs when hydrogen is bonded to a highly electronegative atom like oxygen, nitrogen, or fluorine. These intermolecular forces affect many physical properties, such as melting point, boiling point, and solubility.
Reactivity and Chemical Behavior
Electronegativity influences the reactivity of molecules. Atoms with high electronegativity tend to attract electrons strongly, making them more likely to participate in reactions involving electron transfer or sharing. This is why highly electronegative elements like fluorine and oxygen are highly reactive.
Electronegativity and Beyond: Applications and Extensions
The concept of electronegativity extends beyond its fundamental role in bonding. It plays a vital role in many areas of chemistry and related fields.
Predicting Reaction Mechanisms
Electronegativity helps predict the course of chemical reactions. For example, it can help determine which atom will carry a positive or negative charge in a reaction intermediate or product.
Designing New Materials
Understanding electronegativity is essential in materials science. By carefully selecting elements with specific electronegativities, scientists can design materials with tailored properties, such as high conductivity, high strength, or specific optical characteristics.
Computational Chemistry and Modeling
Electronegativity is incorporated into computational models used to simulate molecular behavior and predict chemical properties. These models play a critical role in drug discovery, materials design, and other areas of scientific research.
Conclusion: A Central Concept in Chemistry
The ability of an atom to attract electrons – its electronegativity – is a fundamental concept with far-reaching consequences. Its influence on bond polarity, molecular properties, reactivity, and even materials science highlights its central role in chemistry. By understanding electronegativity trends and its effects, we can gain deeper insights into the behavior of matter at the molecular level and apply this knowledge to a vast range of scientific and technological applications. From understanding the properties of water to designing novel materials, the implications of electronegativity are profound and continue to shape our understanding of the chemical world.
Latest Posts
Latest Posts
-
What Is 19 25 As A Decimal
May 09, 2025
-
Matter Can Recycle Through The Biosphere Because
May 09, 2025
-
Cells Spend Most Of Their Lives In
May 09, 2025
-
How Are Polyatomic Ions Different From Monatomic Ions
May 09, 2025
-
Which Is Heavier Gold Or Lead
May 09, 2025
Related Post
Thank you for visiting our website which covers about The Ability Of An Atom To Attract Electrons . We hope the information provided has been useful to you. Feel free to contact us if you have any questions or need further assistance. See you next time and don't miss to bookmark.