Predict The Geometry Of Each Interior Atom In Acetic Acid
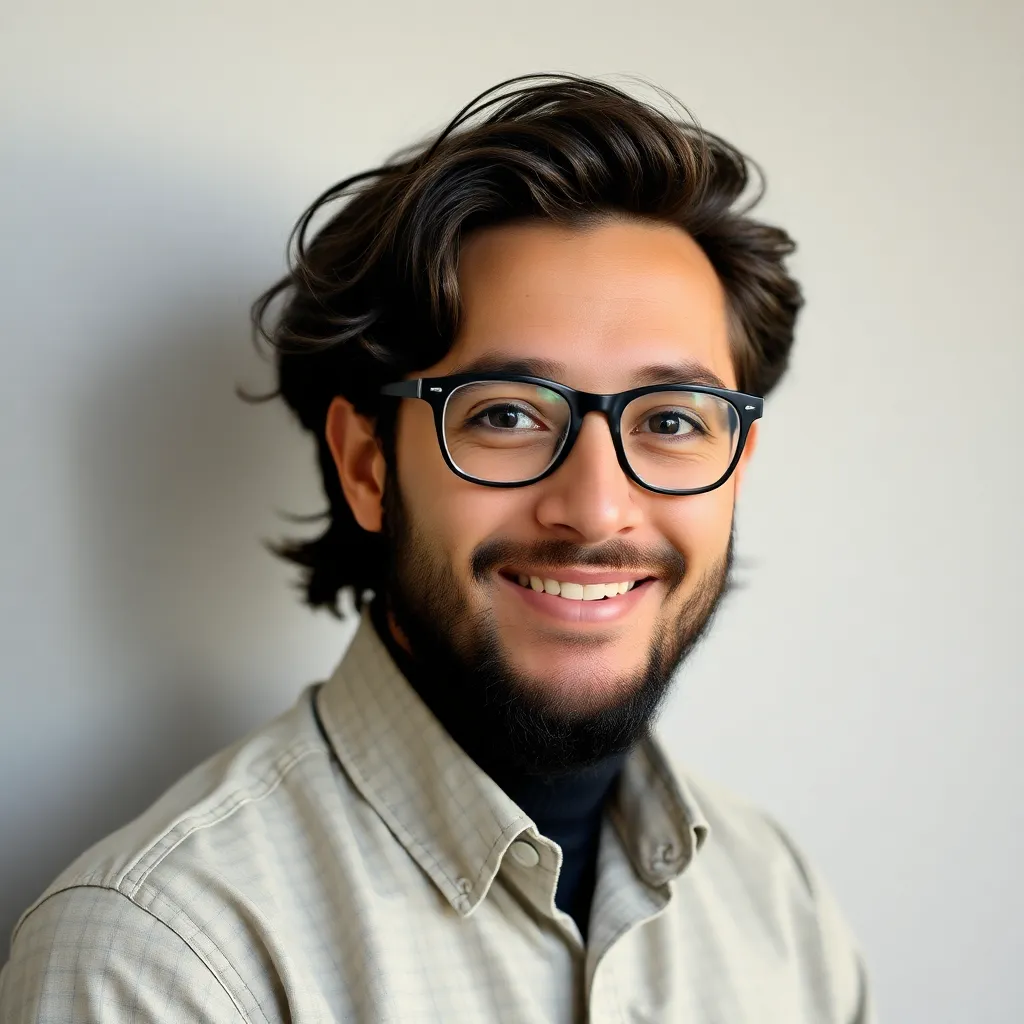
listenit
May 11, 2025 · 5 min read
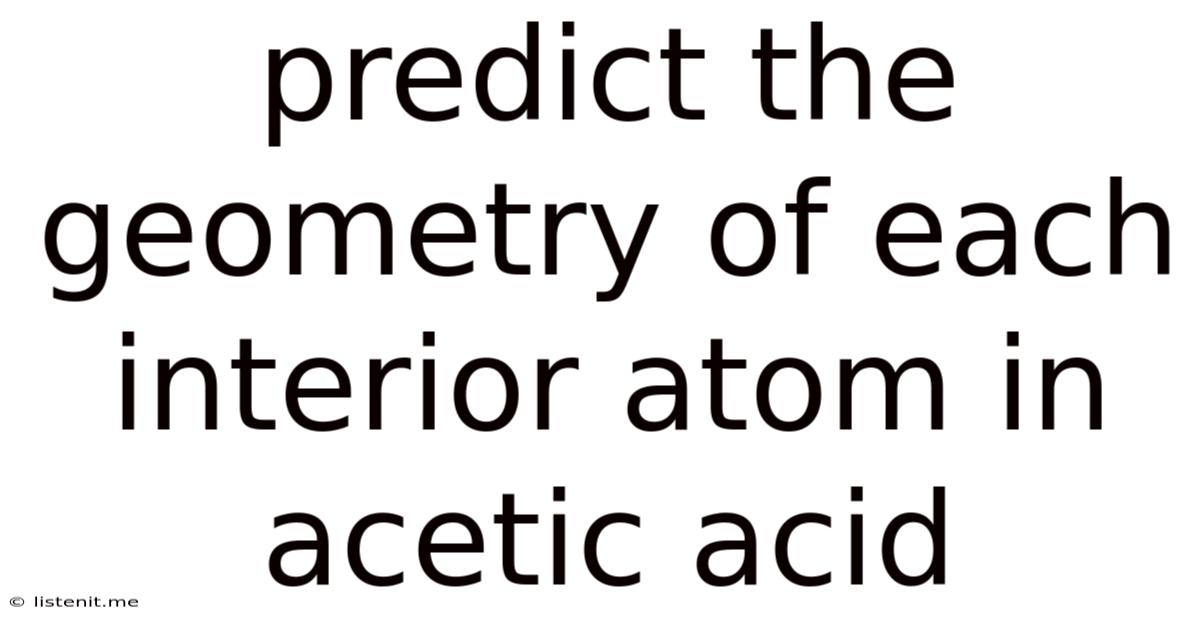
Table of Contents
Predicting the Geometry of Interior Atoms in Acetic Acid: A Comprehensive Guide
Acetic acid, also known as ethanoic acid, is a simple carboxylic acid with the chemical formula CH₃COOH. Understanding its molecular geometry is crucial for comprehending its chemical properties and reactivity. This article will delve into predicting the geometry of each interior atom within the acetic acid molecule using Valence Shell Electron Pair Repulsion (VSEPR) theory and hybrid orbital theory. We will examine the bond angles and overall molecular shape, providing a detailed and comprehensive analysis.
Understanding VSEPR Theory and Hybrid Orbitals
Before diving into the specifics of acetic acid, let's briefly review the fundamental concepts of VSEPR theory and hybrid orbital theory, which are essential for predicting molecular geometry.
VSEPR Theory: The Foundation of Molecular Shape
VSEPR theory postulates that the shape of a molecule is determined by the repulsion between electron pairs in the valence shell of the central atom. These electron pairs can be either bonding pairs (involved in covalent bonds) or lone pairs (non-bonding electron pairs). The electron pairs arrange themselves to minimize repulsion, leading to specific geometric arrangements. The key is to consider all electron pairs, both bonding and lone pairs, when determining the electron-pair geometry. The molecular geometry, however, only considers the positions of the atoms.
Hybrid Orbital Theory: Explaining Bonding
Hybrid orbital theory explains how atomic orbitals combine to form hybrid orbitals that participate in bonding. This is especially crucial for understanding the geometry around atoms with multiple bonds. The hybridization state depends on the number of sigma (σ) bonds and lone pairs surrounding the central atom. Common hybridization states include sp, sp², and sp³.
Predicting the Geometry of Atoms in Acetic Acid
Acetic acid possesses several interior atoms: two carbons, two oxygens, and four hydrogens. Let's analyze the geometry around each of these atoms individually:
Carbon 1 (Methyl Carbon, CH₃)
- Electron Domain Geometry: The carbon atom in the methyl group (CH₃) is bonded to three hydrogen atoms and one carbon atom. This gives it four electron domains (four sigma bonds).
- Hybridization: With four sigma bonds, the carbon atom undergoes sp³ hybridization.
- Molecular Geometry: The four electron domains arrange themselves tetrahedrally to minimize repulsion. Therefore, the molecular geometry around this carbon atom is tetrahedral, with approximate bond angles of 109.5°.
Carbon 2 (Carboxylic Carbon, COOH)
- Electron Domain Geometry: This carbon atom is bonded to one methyl carbon, one hydroxyl oxygen (–OH), and one carbonyl oxygen (=O). It has three sigma bonds and one pi (π) bond. In VSEPR theory, we count each bond as a single electron domain, regardless of whether it is a sigma or pi bond. This gives three electron domains around this carbon atom. Note that the double bond to the oxygen counts as one electron domain.
- Hybridization: With three sigma bonds, this carbon atom undergoes sp² hybridization.
- Molecular Geometry: The three electron domains arrange themselves in a trigonal planar geometry. Therefore, the molecular geometry around this carbon atom is trigonal planar, with approximate bond angles of 120°.
Oxygen 1 (Hydroxyl Oxygen, –OH)
- Electron Domain Geometry: This oxygen atom is bonded to one carbon atom and one hydrogen atom. It also has two lone pairs of electrons. This results in four electron domains.
- Hybridization: With two sigma bonds and two lone pairs, the oxygen atom undergoes sp³ hybridization.
- Molecular Geometry: Although the electron-pair geometry is tetrahedral, the molecular geometry (considering only the positions of the atoms) is bent or V-shaped, with a bond angle slightly less than 109.5° due to the repulsion from the lone pairs.
Oxygen 2 (Carbonyl Oxygen, =O)
- Electron Domain Geometry: This oxygen atom is double-bonded to the carboxylic carbon. It also has two lone pairs of electrons. This gives a total of three electron domains (one double bond counts as one domain).
- Hybridization: With one sigma bond and two lone pairs, this oxygen atom undergoes sp² hybridization.
- Molecular Geometry: The electron domains are arranged in a trigonal planar geometry. The molecular geometry, considering only the atom positions, is bent with a bond angle close to 120 degrees. However, because of the lone pairs and double bond, there is a slightly smaller angle than the ideal 120 degrees from the trigonal planar geometry.
Hydrogens
The hydrogens in the methyl group are all essentially equivalent, each experiencing a tetrahedral geometry from the perspective of the carbon atom they are bonded to. The hydrogen in the hydroxyl group (-OH) experiences a bent geometry due to the sp³ hybridization of the oxygen atom.
Influence of Factors Affecting Bond Angles
While VSEPR theory provides a good prediction of the bond angles, slight deviations can occur due to several factors:
- Lone Pair Repulsion: Lone pairs of electrons occupy more space than bonding pairs, resulting in greater repulsion and compression of the bond angles. This effect is evident in the bent geometry around the hydroxyl oxygen.
- Steric Hindrance: The bulkiness of the substituent groups can cause steric hindrance, leading to slight distortions in the bond angles.
- Resonance: In acetic acid, resonance plays a significant role, particularly in the carboxyl group. The pi electrons in the carbonyl double bond are delocalized, affecting the bond lengths and angles within the carboxyl group. This delocalization contributes to the slightly lower bond angle in the carboxyl group compared to the ideal trigonal planar angle of 120 degrees. This resonance stabilization further influences the overall structure and reactivity of the molecule.
- Hydrogen Bonding: The presence of hydrogen bonding can also subtly influence the bond angles. Hydrogen bonds between acetic acid molecules in solution or solid state will affect the structure. Intermolecular interactions will alter the immediate environment of the individual atoms, impacting the bond angles and overall structure.
Conclusion: A Comprehensive Picture of Acetic Acid Geometry
By applying VSEPR theory and hybrid orbital theory, we have successfully predicted the geometry around each interior atom in acetic acid. While the idealized geometries provide a strong foundation for understanding the molecular structure, it's important to acknowledge the subtle influences of factors like lone pair repulsion, steric effects, resonance, and hydrogen bonding which cause slight deviations from these ideal angles. Understanding these deviations provides a more complete and accurate picture of the acetic acid molecule’s three-dimensional structure and helps explain its reactivity and physical properties. The combination of theoretical predictions and experimental observations (e.g., X-ray crystallography) gives the most complete and nuanced perspective on the actual geometry of this important molecule.
Latest Posts
Latest Posts
-
What Percent Of 300 Is 90
May 11, 2025
-
What Do Plant Animal And Bacterial Cells Have In Common
May 11, 2025
-
Moment Generating Function Of Chi Square Distribution
May 11, 2025
-
What Are Valence Electrons Why Are They Important
May 11, 2025
-
How Was The Periodic Table First Arranged
May 11, 2025
Related Post
Thank you for visiting our website which covers about Predict The Geometry Of Each Interior Atom In Acetic Acid . We hope the information provided has been useful to you. Feel free to contact us if you have any questions or need further assistance. See you next time and don't miss to bookmark.