Number Of Covalent Bonds In Carbon
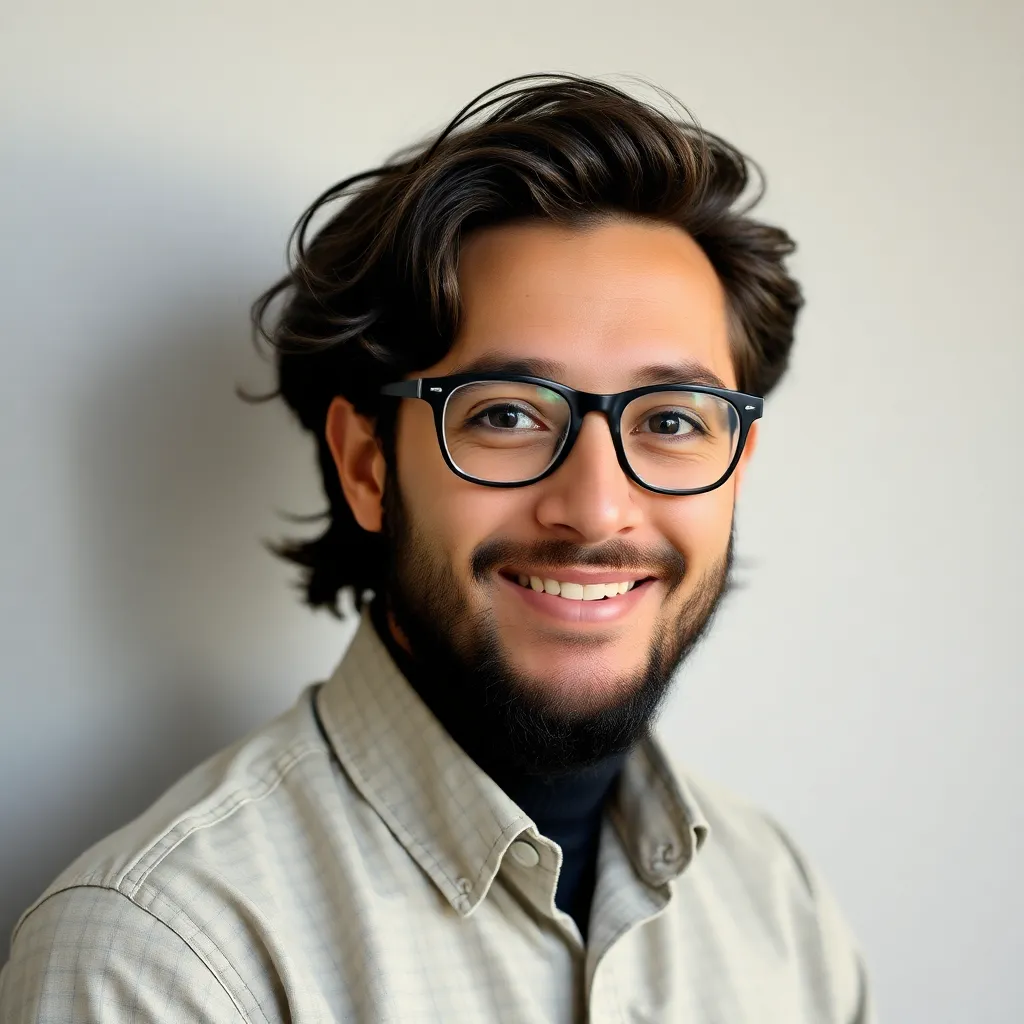
listenit
May 12, 2025 · 7 min read
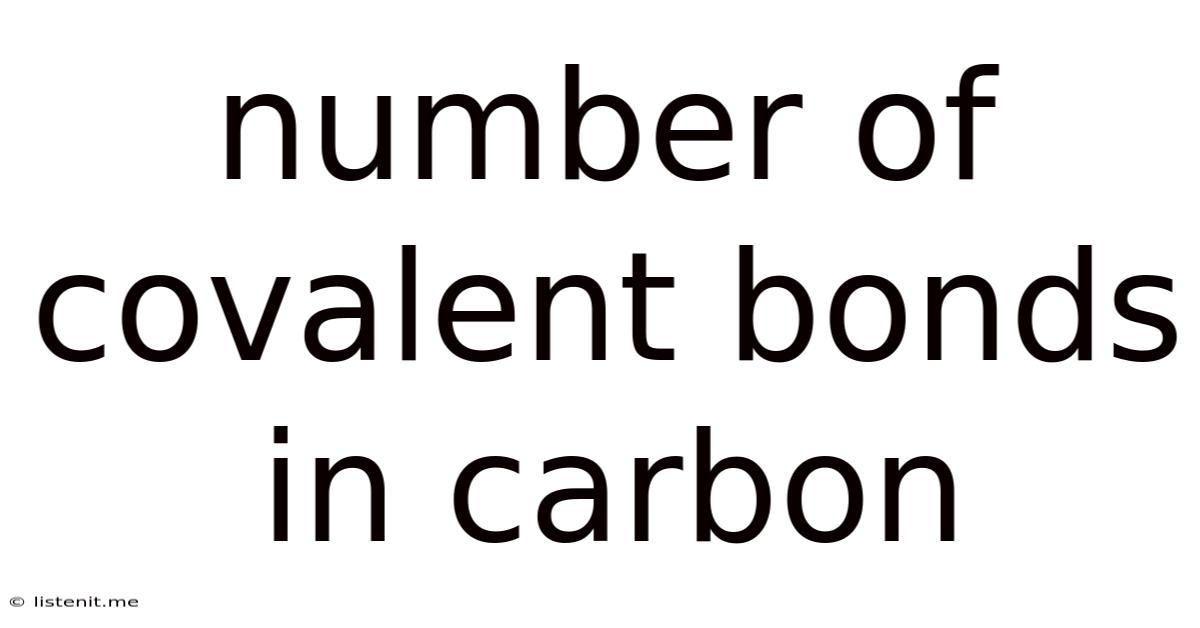
Table of Contents
The Curious Case of Carbon: Unveiling the Number of Covalent Bonds
Carbon, the backbone of life and a cornerstone of organic chemistry, exhibits a remarkable versatility in its bonding behavior. Unlike many other elements, carbon consistently forms four covalent bonds. This characteristic is fundamental to the incredible diversity of organic molecules found in nature and synthesized in laboratories. This article delves deep into the reasons behind carbon's tetravalency, exploring its electronic configuration, hybridization, and the implications for the vast array of carbon-based compounds. We will also examine exceptions and nuances to this rule, highlighting the fascinating complexity of carbon chemistry.
Understanding Carbon's Electronic Configuration: The Key to Tetravalency
The secret to carbon's penchant for four covalent bonds lies within its electronic structure. A neutral carbon atom possesses six electrons, distributed across two electron shells. The first shell contains two electrons, while the second, or valence shell, holds the remaining four. This electronic configuration is denoted as 1s²2s²2p².
It's crucial to understand that atoms strive for a stable, low-energy state, often achieved by filling their valence shell with eight electrons (the octet rule). Carbon, with only four valence electrons, needs four more to attain this stable octet. The most efficient way to achieve this is by forming four covalent bonds—sharing one electron with each of four other atoms. This explains carbon's tetravalency, its unique ability to form four stable covalent bonds.
The Role of Hybridization: Shaping Carbon's Bonding Geometry
While the simple electronic configuration explains the number of bonds, it doesn't fully explain the geometry of these bonds. To understand this, we need to delve into the concept of orbital hybridization.
In its ground state, carbon's valence electrons are arranged as one 2s electron pair and two 2p electrons (one in each of the 2px and 2py orbitals, leaving the 2pz orbital empty). This arrangement doesn't predict the tetrahedral geometry observed in many carbon compounds like methane (CH₄).
To address this discrepancy, the concept of hybridization is introduced. In carbon's case, one 2s electron is promoted to the empty 2pz orbital, resulting in four unpaired electrons. These four orbitals then hybridize—mixing together—to form four equivalent sp³ hybrid orbitals. These sp³ orbitals are oriented in a tetrahedral arrangement, maximizing the distance between electron pairs and minimizing repulsion. This tetrahedral arrangement leads to bond angles of approximately 109.5 degrees in molecules like methane.
This hybridization is crucial because it facilitates the formation of four strong sigma (σ) bonds, each utilizing one sp³ hybrid orbital from the carbon atom and one orbital from the bonded atom. The strength and stability of these σ bonds are essential to the stability of countless organic molecules.
Exploring Different Hybridization States and Their Implications
While sp³ hybridization is the most common for carbon, leading to four single bonds, carbon can also exhibit other hybridization states, influencing the number and type of bonds it forms:
-
sp Hybridization: In molecules containing triple bonds, like ethyne (acetylene, C₂H₂), carbon undergoes sp hybridization. One 2s orbital combines with one 2p orbital, producing two sp hybrid orbitals oriented 180 degrees apart. These form two sigma bonds. The remaining two unhybridized 2p orbitals form two pi (π) bonds, resulting in a total of four bonds: two sigma and two pi bonds.
-
sp² Hybridization: This hybridization occurs in molecules containing double bonds, like ethene (ethylene, C₂H₄). One 2s orbital combines with two 2p orbitals to create three sp² hybrid orbitals arranged in a trigonal planar geometry (120-degree bond angles). These form three sigma bonds. The remaining unhybridized 2p orbital forms one pi (π) bond, giving a total of four bonds per carbon atom: three sigma and one pi bond.
The Impact on Molecular Geometry and Properties: A Diverse Landscape
The hybridization state profoundly impacts the molecular geometry and, consequently, the physical and chemical properties of organic molecules. The tetrahedral geometry of sp³ hybridized carbon in alkanes, for instance, contrasts sharply with the linear geometry of sp hybridized carbon in alkynes and the trigonal planar geometry of sp² hybridized carbon in alkenes. These differences influence factors such as melting and boiling points, reactivity, and solubility.
The presence of pi (π) bonds in sp² and sp hybridized carbons also plays a crucial role. Pi bonds are less strong than sigma bonds but significantly impact a molecule's reactivity. The delocalized pi electrons in conjugated systems (alternating single and multiple bonds) contribute to properties like enhanced stability and unique spectral characteristics.
Exceptions and Nuances: When Carbon Doesn't Always Form Four Bonds
While the rule of four bonds is prevalent for carbon, some exceptions exist, primarily in reactive intermediates and unusual bonding scenarios:
-
Carbenes: These are highly reactive neutral molecules containing a divalent carbon atom with two unshared electrons. The carbon in a carbene forms only two bonds, defying the typical tetravalency. Their high reactivity stems from the presence of the two unpaired electrons, making them crucial intermediates in many organic reactions.
-
Carbon Radicals: These species possess an unpaired electron on a carbon atom. Depending on their structure and environment, carbon radicals can form three bonds, leaving one electron unpaired. Their high reactivity makes them important in polymerization reactions and other free-radical processes.
-
Carbocations and Carbanions: These are charged species. Carbocations have a positively charged carbon atom with only three bonds, while carbanions possess a negatively charged carbon atom with three bonds and a lone pair of electrons. Both are highly reactive intermediates frequently involved in organic reactions.
Beyond Simple Molecules: The Complexity of Carbon Chemistry
The concept of carbon forming four bonds becomes increasingly complex as we move beyond simple hydrocarbons. In larger molecules, particularly those containing functional groups (e.g., alcohols, ketones, carboxylic acids), the bonding patterns around the carbon atoms are influenced by the presence of heteroatoms (atoms other than carbon and hydrogen) and their electron-donating or electron-withdrawing capabilities.
These heteroatoms can significantly influence the electron density around carbon atoms, affecting bond lengths, bond angles, and overall molecular properties. Understanding these intricacies requires a deeper understanding of molecular orbital theory and advanced concepts in organic chemistry.
The Significance of Carbon's Tetravalency: The Foundation of Organic Chemistry and Life Itself
The unique ability of carbon to form four strong covalent bonds is a defining feature that underpins the remarkable diversity of organic molecules. This tetravalency, coupled with carbon's ability to form chains, rings, and branched structures, allows for an almost limitless number of organic compounds, forming the basis for the complexity of life.
From Simple to Complex: The Building Blocks of Life
The four bonds allow carbon to act as a versatile building block, linking together to form long chains (polymers), creating complex rings, and branching out in diverse patterns. This forms the basis for the construction of proteins, carbohydrates, lipids, and nucleic acids—the fundamental components of all living organisms.
Applications in Materials Science and Beyond: Carbon's Enduring Legacy
The unparalleled versatility of carbon bonding isn't limited to biological systems. Carbon's ability to form diverse structures underlies the vast array of synthetic materials, from polymers (plastics) to carbon fibers and graphene—a single layer of carbon atoms arranged in a hexagonal lattice, possessing extraordinary strength and electrical conductivity. Carbon nanotubes, cylindrical structures composed of carbon atoms, exhibit exceptional mechanical and electrical properties, finding applications in various technological fields.
Conclusion: A Journey into the Heart of Carbon Chemistry
The consistent formation of four covalent bonds by carbon is not simply a rule; it is a fundamental principle that underpins the rich tapestry of organic chemistry and the very essence of life itself. Understanding the electronic configuration, hybridization, and the nuanced exceptions to this rule unveils the intricate complexities that make carbon such a fascinating and essential element. This exploration highlights the power of fundamental concepts in chemistry and the incredible diversity born from the simple yet profoundly significant ability of a carbon atom to form four covalent bonds. From simple hydrocarbons to the complex molecules of life, carbon's tetravalency remains a testament to nature's elegant design and boundless creativity. The continued exploration of carbon's multifaceted chemistry promises further exciting discoveries and technological advancements in the years to come.
Latest Posts
Related Post
Thank you for visiting our website which covers about Number Of Covalent Bonds In Carbon . We hope the information provided has been useful to you. Feel free to contact us if you have any questions or need further assistance. See you next time and don't miss to bookmark.