Nucleic Acid Polymers Are Made Up Of
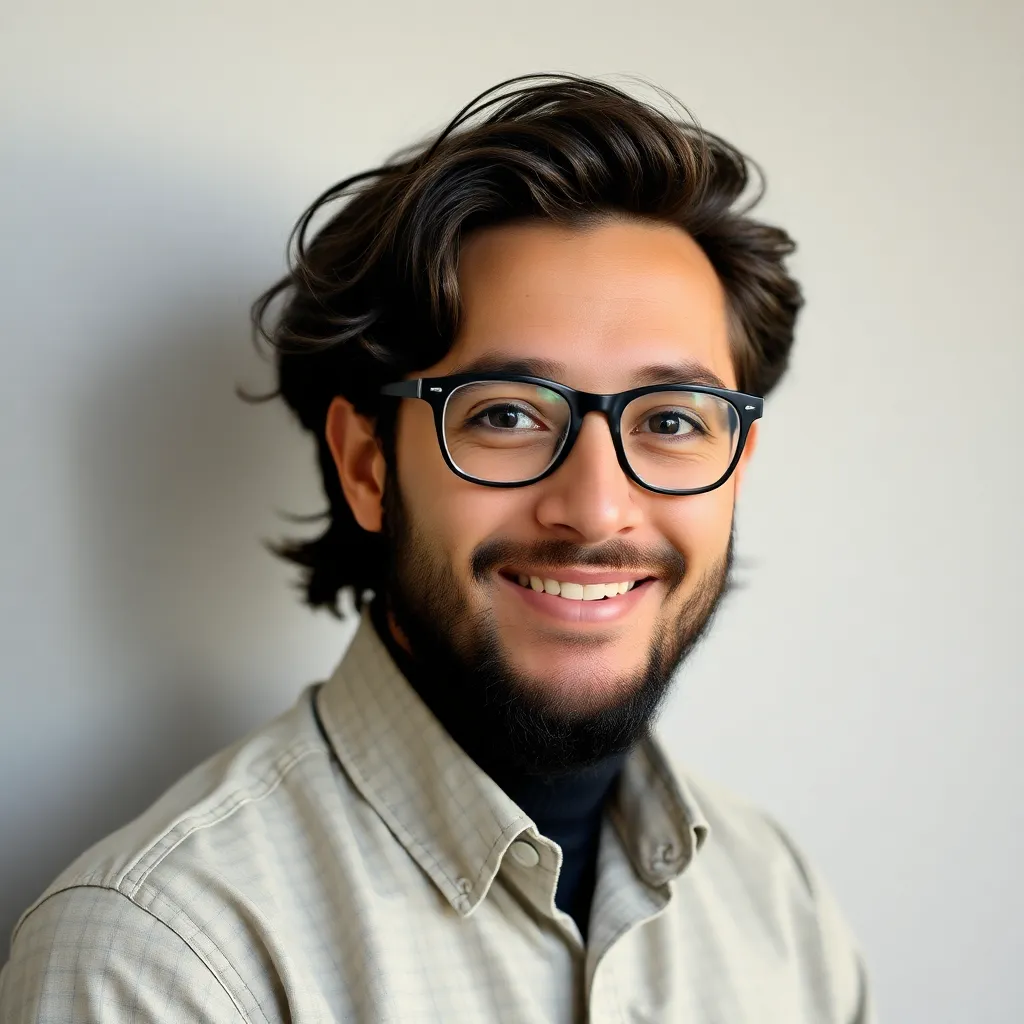
listenit
May 10, 2025 · 6 min read
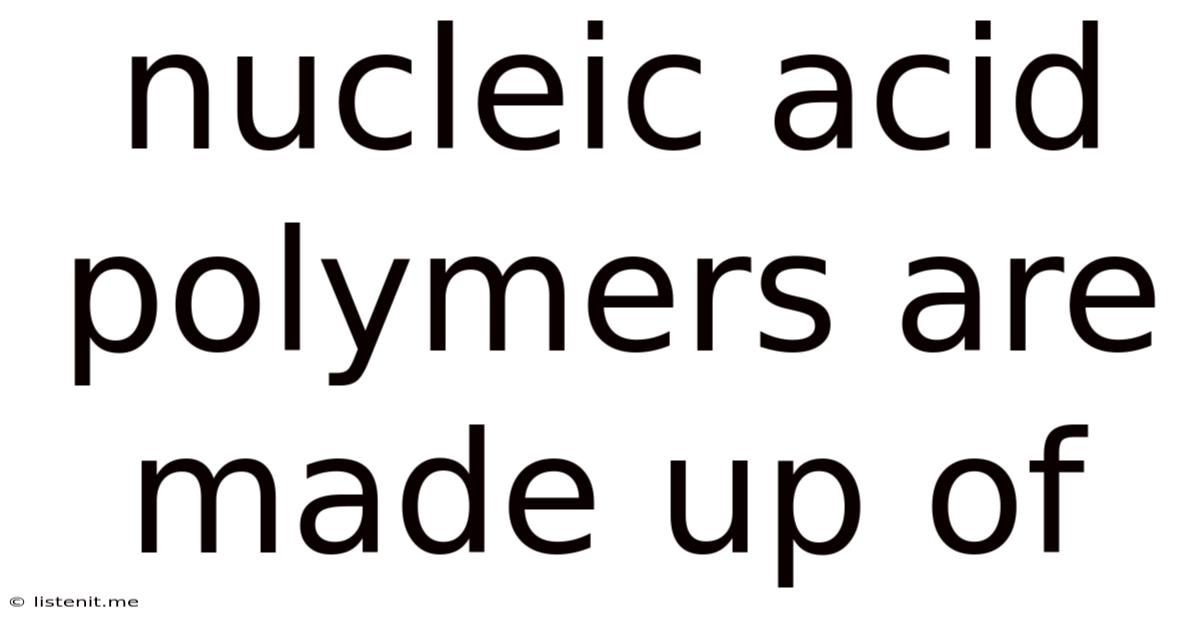
Table of Contents
Nucleic Acid Polymers: A Deep Dive into Their Composition and Structure
Nucleic acids are fundamental macromolecules of life, responsible for storing, transmitting, and expressing genetic information. These vital biopolymers come in two primary forms: deoxyribonucleic acid (DNA) and ribonucleic acid (RNA). Understanding the building blocks and intricate structure of these polymers is crucial to comprehending the mechanisms of heredity, gene expression, and numerous cellular processes. This article delves into the detailed composition of nucleic acid polymers, exploring their fundamental units, the bonds that link them, and the higher-order structures they adopt.
The Monomers: Nucleotides – The Building Blocks of Life
Both DNA and RNA are linear polymers composed of repeating monomeric units called nucleotides. Each nucleotide comprises three essential components:
-
A Pentose Sugar: A five-carbon sugar forms the backbone of the nucleotide. In DNA, this sugar is deoxyribose, while in RNA, it's ribose. The crucial difference lies in the presence of a hydroxyl (-OH) group on the 2' carbon of ribose, absent in deoxyribose. This seemingly minor difference has profound implications for the stability and function of the two nucleic acids. Ribose's extra hydroxyl group makes RNA more susceptible to hydrolysis, contributing to its generally shorter lifespan compared to DNA.
-
A Nitrogenous Base: This is a heterocyclic aromatic ring structure containing nitrogen atoms. There are five main nitrogenous bases found in nucleic acids:
- Adenine (A) and Guanine (G) are purines, characterized by a double-ring structure.
- Cytosine (C), Thymine (T), and Uracil (U) are pyrimidines, possessing a single-ring structure.
- Thymine (T) is found exclusively in DNA, while Uracil (U) is found only in RNA. Both T and U are similar in structure, differing only by a methyl group.
-
A Phosphate Group: This negatively charged group, (PO₄³⁻), is crucial for linking nucleotides together to form the polynucleotide chain. The phosphate group connects the 5' carbon of one sugar to the 3' carbon of the next sugar, creating a phosphodiester bond. This directionality, from 5' to 3', is essential for understanding DNA replication and transcription.
The Phosphodiester Bond: Linking Nucleotides into Chains
The phosphodiester bond is the key linkage that unites nucleotides, forming the backbone of nucleic acid polymers. This bond is formed through a dehydration reaction between the 5'-phosphate group of one nucleotide and the 3'-hydroxyl group of another nucleotide. This process releases a water molecule and results in a strong, stable covalent bond.
The continuous phosphodiester backbone exhibits a remarkable property: directionality. The chain has a 5' end (with a free phosphate group) and a 3' end (with a free hydroxyl group). This directionality is critical for various enzymatic processes, such as DNA replication and RNA transcription, where enzymes only work in one specific direction along the nucleic acid chain.
Primary Structure: The Sequence of Nucleotides
The primary structure of a nucleic acid refers to the linear sequence of nucleotides linked together by phosphodiester bonds. This sequence determines the genetic information encoded within the molecule. For instance, the sequence of bases in DNA determines the order of amino acids in proteins. The primary structure is dictated by the specific order of nucleotides, which are not random but follow a precise sequence determined by the genetic code. Variations in this sequence account for the enormous diversity of genetic information found in all living organisms.
Secondary Structure: The Double Helix of DNA and RNA Folding
The secondary structure refers to the three-dimensional arrangement of the polynucleotide chain. This is where the iconic double helix of DNA comes into play. In DNA, two polynucleotide chains, running antiparallel (one 5' to 3', the other 3' to 5'), twist around each other to form a right-handed double helix. The two strands are held together by hydrogen bonds between complementary base pairs: Adenine (A) pairs with Thymine (T) via two hydrogen bonds, and Guanine (G) pairs with Cytosine (C) via three hydrogen bonds. This base pairing is the foundation of DNA's remarkable ability to replicate and store genetic information faithfully.
RNA, in contrast, usually exists as a single-stranded molecule. However, RNA molecules can fold into complex secondary structures through intramolecular base pairing. These structures often include hairpin loops, stem-loops, and other intricate formations stabilized by hydrogen bonds between complementary bases. These secondary structures are crucial for RNA's diverse functions, such as messenger RNA (mRNA) carrying genetic information, transfer RNA (tRNA) mediating protein synthesis, and ribosomal RNA (rRNA) forming ribosomes.
Tertiary and Quaternary Structure: Higher Order Organization
While the double helix is the defining secondary structure of DNA, it doesn't tell the whole story. DNA molecules further organize into higher-order structures, including:
-
Supercoiling: The double helix can twist upon itself, forming supercoils. This compacting process is essential for fitting the vast amount of DNA into the cell nucleus. Enzymes called topoisomerases regulate supercoiling.
-
Chromatin: In eukaryotic cells, DNA is packaged with proteins called histones to form chromatin. Histones wrap DNA around them, further compacting the molecule and regulating gene expression. Chromatin then organizes into chromosomes during cell division.
Similarly, RNA molecules can also adopt complex tertiary and quaternary structures. For example, tRNA molecules fold into a characteristic cloverleaf shape, stabilized by hydrogen bonds and other interactions. This specific three-dimensional structure is crucial for tRNA's role in translating mRNA into proteins. Ribosomes, composed of rRNA and proteins, represent a sophisticated quaternary structure, forming a complex machinery for protein synthesis.
Differences between DNA and RNA Structure and Function
While both DNA and RNA are nucleic acid polymers built from nucleotides, several key differences distinguish them:
Feature | DNA | RNA |
---|---|---|
Sugar | Deoxyribose | Ribose |
Bases | Adenine, Guanine, Cytosine, Thymine | Adenine, Guanine, Cytosine, Uracil |
Structure | Typically double-stranded helix | Typically single-stranded, can fold |
Stability | More stable due to lack of 2'-OH group | Less stable due to presence of 2'-OH group |
Function | Long-term storage of genetic information | Diverse functions including gene expression |
The Significance of Nucleic Acid Structure in Biological Processes
The intricate structures of nucleic acids are intrinsically linked to their diverse functions within the cell. The double-helix structure of DNA, with its complementary base pairing, provides a mechanism for accurate replication, allowing for the faithful transmission of genetic information from one generation to the next. The specific sequence of nucleotides in DNA dictates the sequence of amino acids in proteins through the process of transcription and translation. The various secondary and tertiary structures adopted by RNA molecules are essential for their roles in gene expression, protein synthesis, and other cellular processes.
Conclusion: A Dynamic World of Nucleic Acid Polymers
The study of nucleic acid polymers is a journey into the heart of life itself. Understanding the composition, structure, and functions of DNA and RNA is crucial for comprehending the fundamental mechanisms that drive heredity, gene expression, and the intricate workings of cells. From the simple nucleotide building blocks to the complex three-dimensional structures, nucleic acids exhibit a remarkable level of organization and complexity, underlying their vital roles in maintaining the continuity of life. Continued research in this field promises to unlock even more secrets about the fascinating world of these biopolymers and their profound influence on all aspects of biology.
Latest Posts
Latest Posts
-
Find The Lcm Of 12 And 15
May 10, 2025
-
Ground State Electron Configuration Of Cr
May 10, 2025
-
How Many Protons Are In Ne
May 10, 2025
-
How To Solve Inequalities With Absolute Values On Both Sides
May 10, 2025
-
The Quotient Of 5 And A Number
May 10, 2025
Related Post
Thank you for visiting our website which covers about Nucleic Acid Polymers Are Made Up Of . We hope the information provided has been useful to you. Feel free to contact us if you have any questions or need further assistance. See you next time and don't miss to bookmark.