N2 Electron Geometry And Molecular Geometry
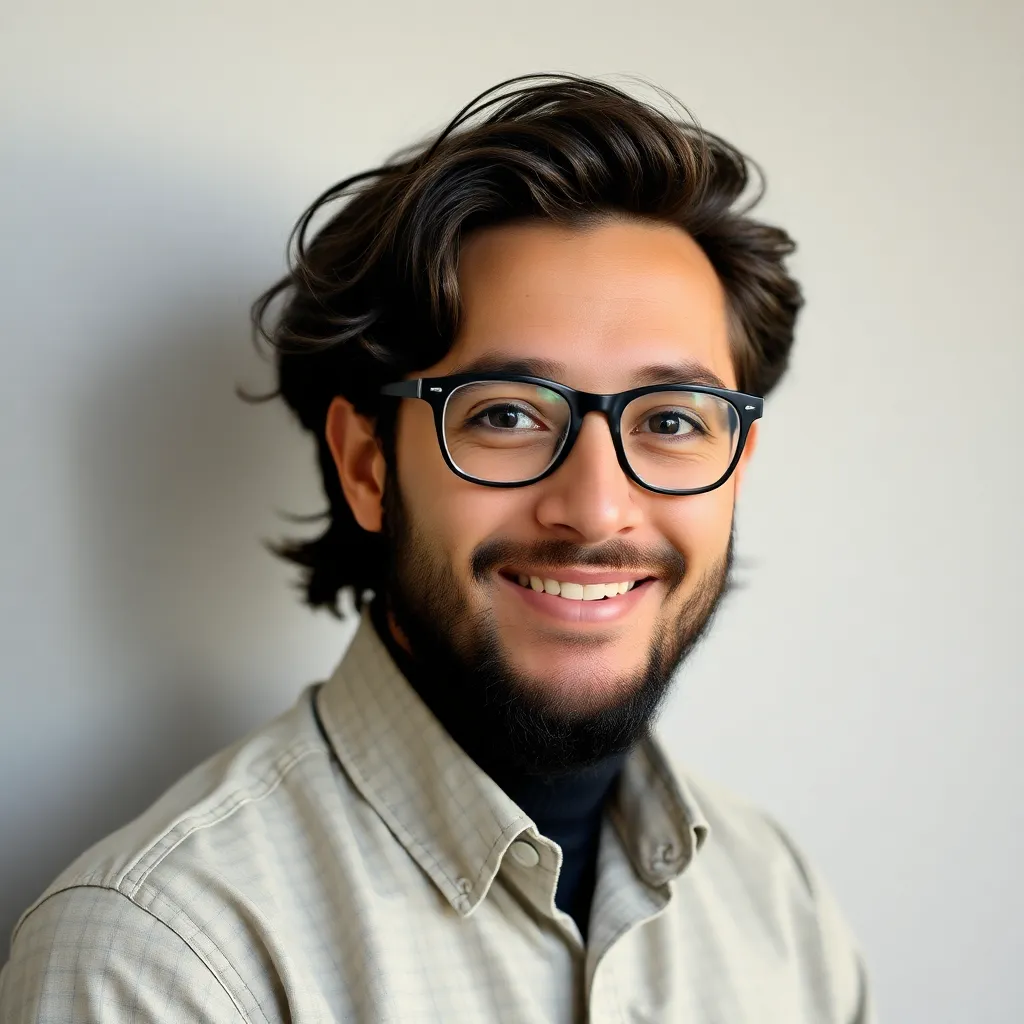
listenit
Apr 08, 2025 · 6 min read

Table of Contents
N₂ Electron Geometry and Molecular Geometry: A Deep Dive
Understanding the shapes of molecules is crucial in chemistry. It dictates their properties, reactivity, and interactions. This in-depth article explores the electron geometry and molecular geometry of nitrogen gas (N₂), a fundamental molecule with a deceptively simple structure. We will delve into the concepts of Valence Shell Electron Pair Repulsion (VSEPR) theory and apply it to unravel the intricacies of N₂'s geometry. This explanation will be thorough, aiming to provide a strong foundational understanding for students and enthusiasts alike.
Understanding VSEPR Theory: The Foundation of Molecular Geometry
Before diving into the specifics of N₂, let's lay the groundwork by understanding the Valence Shell Electron Pair Repulsion (VSEPR) theory. This cornerstone of chemical bonding theory postulates that the electron pairs surrounding a central atom will arrange themselves to minimize repulsion, leading to specific geometric structures. The repulsion between electron pairs—both bonding and lone pairs—drives the overall shape of the molecule. The strength of repulsion follows this order:
- Lone pair-lone pair repulsion > lone pair-bonding pair repulsion > bonding pair-bonding pair repulsion
This means that lone pairs have a stronger influence on the molecular geometry than bonding pairs.
Key Terms: Electron Geometry vs. Molecular Geometry
It's crucial to distinguish between electron geometry and molecular geometry.
-
Electron geometry describes the arrangement of all electron pairs (both bonding and lone pairs) around the central atom. It considers the spatial distribution of all electron clouds.
-
Molecular geometry describes the arrangement of only the atoms in the molecule. It focuses solely on the positions of the nuclei and ignores the lone pairs, even though their presence significantly affects the molecular shape.
Analyzing the Nitrogen Molecule (N₂): A Linear Structure
Nitrogen gas (N₂) is a diatomic molecule, meaning it's composed of two nitrogen atoms. Each nitrogen atom has five valence electrons. To achieve a stable octet, they share three electron pairs in a triple bond:
N≡N
Let's apply VSEPR theory to analyze its geometry:
1. Determining the Electron Geometry of N₂
Each nitrogen atom is surrounded by two electron domains: one is a lone pair and three electrons are involved in bonding to the other nitrogen atom. In N2, however, since both nitrogen atoms are identical and share the same bonding situation, there's really only one central atom to consider. The presence of a triple bond results in three bonding electron pairs surrounding each nitrogen atom. According to VSEPR theory, three bonding electron pairs will arrange themselves linearly to minimize repulsion. Therefore, the electron geometry of N₂ is linear.
2. Determining the Molecular Geometry of N₂
Since there are no lone pairs on either nitrogen atom, the molecular geometry is identical to the electron geometry. The two nitrogen atoms are arranged in a straight line. Therefore, the molecular geometry of N₂ is also linear.
Visualizing the Linear Geometry of N₂
Imagine drawing a straight line. That line perfectly represents the linear arrangement of the two nitrogen atoms in N₂. There's no deviation from this straight line because there are no lone pairs to cause bending or distortion. The triple bond efficiently overlaps to create a strong and stable bond, leading to this simple and highly symmetrical structure. This linear structure influences several key properties of N₂, including its non-polar nature and low reactivity under normal conditions.
Consequences of N₂'s Linear Geometry
The linear geometry of N₂ has far-reaching implications for its chemical and physical properties:
-
Non-polarity: Because the molecule is symmetrical, the bond dipoles of the N-N triple bond cancel each other out, resulting in a non-polar molecule. This significantly impacts its solubility in polar solvents.
-
High Bond Energy: The triple bond in N₂ is exceptionally strong, requiring a substantial amount of energy to break. This contributes to nitrogen's relative inertness at room temperature and explains its prevalence in the atmosphere.
-
Low Reactivity: The strong triple bond makes N₂ relatively unreactive under normal conditions. This inertness is crucial for its role as a major component of Earth's atmosphere. However, under specific conditions (such as high temperatures and pressures, or in the presence of catalysts), nitrogen can react to form important compounds like ammonia (NH₃).
-
Physical State: At standard temperature and pressure, N₂ exists as a gas due to the relatively weak intermolecular forces between the non-polar molecules.
Comparing N₂ to Other Molecules: Illustrating VSEPR Principles
To solidify our understanding, let's compare N₂ to other molecules and illustrate the impact of lone pairs on molecular geometry. Consider these examples:
-
CO₂ (Carbon Dioxide): Similar to N₂, CO₂ has a linear electron geometry and a linear molecular geometry. Carbon forms double bonds with each oxygen atom, leading to the absence of lone pairs on the central carbon atom.
-
H₂O (Water): Water has a tetrahedral electron geometry (due to four electron pairs around the oxygen atom), but a bent molecular geometry. The presence of two lone pairs on the oxygen atom repels the bonding pairs, resulting in a bent shape instead of a linear one.
-
NH₃ (Ammonia): Ammonia has a tetrahedral electron geometry (four electron pairs around the nitrogen atom) and a trigonal pyramidal molecular geometry. One lone pair on the nitrogen atom pushes the three bonding pairs closer together, resulting in a pyramidal shape.
These examples highlight that while electron geometry considers all electron pairs, molecular geometry focuses only on the arrangement of atoms, with lone pairs influencing the overall shape.
Advanced Concepts and Applications
The fundamental understanding of N₂'s geometry allows us to delve into more advanced concepts:
-
Hybridization: The nitrogen atoms in N₂ undergo sp hybridization, where one s orbital and one p orbital combine to form two sp hybrid orbitals involved in the sigma bonds. The remaining two p orbitals on each nitrogen atom form two pi bonds, completing the triple bond.
-
Bond Order and Length: The triple bond in N₂ results in a high bond order (3) and a short bond length, contributing to the molecule's strength and stability.
-
Spectroscopy: The vibrational and rotational spectra of N₂ can be analyzed to confirm its linear structure and provide detailed information about its bond strength and other properties.
Conclusion: The Importance of Understanding Molecular Geometry
The linear electron geometry and molecular geometry of N₂ aren't just abstract concepts; they are fundamental to understanding its chemical and physical behavior. This simple yet crucial example showcases the power of VSEPR theory in predicting and explaining the three-dimensional structures of molecules, paving the way for deeper insights into their properties and reactivity. By understanding the interplay between bonding electrons and lone pairs, we gain a powerful tool for analyzing and predicting the behavior of a vast range of molecules, from simple diatomic gases to complex biological macromolecules. The principles discussed here form the foundation for further exploration in organic, inorganic, and physical chemistry.
Latest Posts
Latest Posts
-
What Do The Roman Numerals In A Cations Name Indicate
Apr 17, 2025
-
Find The Values Of Xy And Z
Apr 17, 2025
-
What Is The Correct Lewis Structure For N2
Apr 17, 2025
-
Show That The Point Is On The Unit Circle
Apr 17, 2025
-
Derivative Of Sec X Tan X
Apr 17, 2025
Related Post
Thank you for visiting our website which covers about N2 Electron Geometry And Molecular Geometry . We hope the information provided has been useful to you. Feel free to contact us if you have any questions or need further assistance. See you next time and don't miss to bookmark.