Molecular Dynamics Simulations Show That The Structure Of Proteins
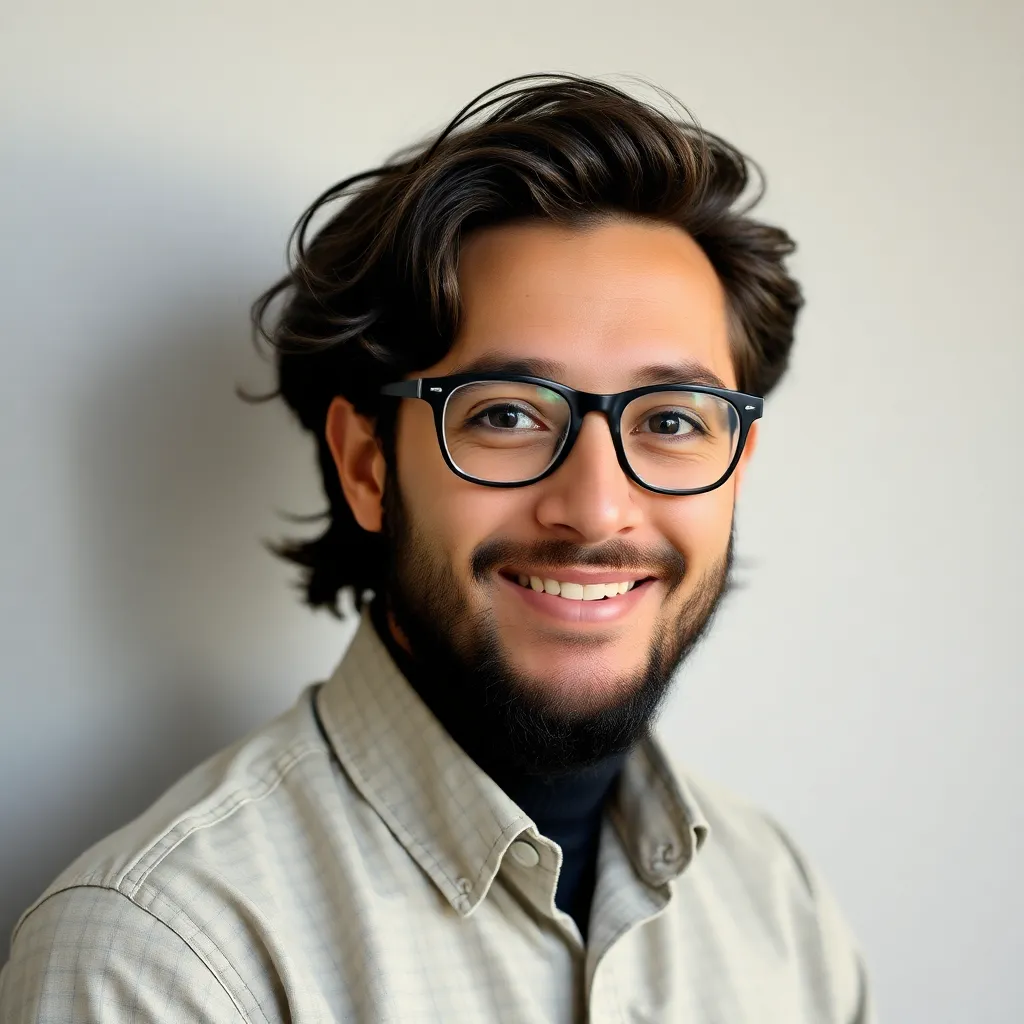
listenit
May 27, 2025 · 6 min read
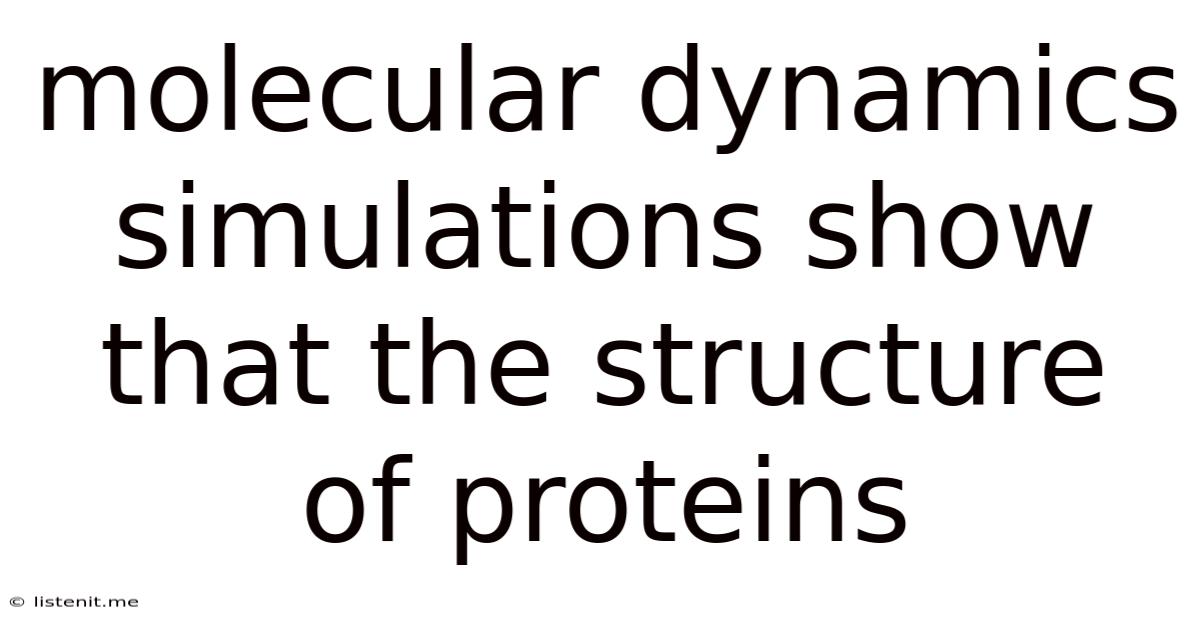
Table of Contents
Molecular Dynamics Simulations: Unveiling the Dynamic Nature of Protein Structure
Proteins, the workhorses of biological systems, are incredibly complex molecules whose functions are intimately tied to their three-dimensional structures. Understanding this structure-function relationship is crucial for advancements in medicine, biotechnology, and materials science. While experimental techniques like X-ray crystallography and NMR spectroscopy provide valuable static snapshots of protein structures, they often fail to capture the dynamic nature of these molecules. This is where molecular dynamics (MD) simulations come in, offering a powerful computational approach to explore the intricate dance of atoms within proteins and reveal their conformational landscapes.
What are Molecular Dynamics Simulations?
Molecular dynamics simulations are computational methods that use classical mechanics to model the movement of atoms and molecules over time. By applying Newton's laws of motion, MD simulations track the changes in the positions and velocities of atoms in a system based on the forces acting upon them. These forces are primarily derived from interatomic potentials, which describe the energy landscape of the system and dictate how atoms interact with each other.
Commonly used force fields, such as AMBER, CHARMM, and GROMACS, parameterize these interactions, including bonded (bonds, angles, dihedrals) and non-bonded (van der Waals forces, electrostatic interactions) terms. These force fields, while approximations of reality, are continually refined to improve accuracy and predictive power.
The simulation proceeds by numerically integrating Newton's equations of motion, typically using algorithms like the Verlet integrator, to generate a trajectory of atomic positions and velocities over a defined time period, often ranging from nanoseconds to microseconds, depending on computational resources and the system's complexity. This trajectory provides a wealth of information about the protein's dynamics.
MD Simulations and Protein Structure: Beyond Static Images
Traditional structural biology techniques, while powerful, provide only a static view of a protein. They capture a single, often averaged, conformation of the protein. However, proteins are inherently dynamic; they constantly fluctuate, adopting various conformations, and these fluctuations are crucial for their function.
MD simulations offer an unparalleled view into this dynamism. By generating trajectories of atomic movements, they allow researchers to:
1. Visualize Protein Flexibility:
MD simulations reveal the flexibility of different regions within a protein. Some regions might exhibit large conformational changes, while others remain relatively rigid. This information is crucial for understanding how proteins bind to ligands, undergo conformational transitions, and interact with other molecules. Identifying flexible loops and hinges is paramount in drug design, allowing for the targeting of specific sites for effective inhibitor binding.
2. Study Protein Folding and Unfolding:
Protein folding, the process by which a linear polypeptide chain acquires its unique three-dimensional structure, is a fundamental biological process. MD simulations, especially those employing enhanced sampling techniques like metadynamics or replica exchange, can shed light on the mechanisms of protein folding and unfolding. They can unveil intermediate states and energy barriers, providing insights into the folding pathways and potential bottlenecks.
3. Investigate Protein-Ligand Interactions:
Understanding how proteins interact with ligands (e.g., drugs, substrates) is critical in drug discovery and enzyme engineering. MD simulations can model these interactions directly, revealing the binding modes, the strength of binding, and the key residues involved in the interaction. This information can be used to design more potent and selective drugs.
4. Analyze Conformational Ensembles:
MD simulations generate a large ensemble of protein conformations, representing the range of structures the protein can adopt under specific conditions. Analyzing this ensemble allows researchers to determine the most populated conformations, quantify the conformational flexibility, and characterize the transitions between different states. This is crucial for understanding the protein’s functional landscape.
Enhancing the Power of MD Simulations: Advanced Techniques
Standard MD simulations, while insightful, are limited by computational constraints. To overcome these limitations and access longer timescales and rarer events, several advanced techniques have been developed:
1. Enhanced Sampling Methods:
Methods such as metadynamics, replica exchange molecular dynamics (REMD), and umbrella sampling enhance the exploration of the conformational space by overcoming high energy barriers that hinder standard MD simulations. These techniques allow researchers to observe rare events and transition states, providing a more comprehensive understanding of protein dynamics.
2. Coarse-Grained Models:
Coarse-grained models represent groups of atoms as single interaction sites, reducing the computational cost significantly. While losing some atomic detail, these models allow simulations to access longer timescales, enabling the study of large-scale conformational changes and processes like protein aggregation.
3. Hybrid Quantum Mechanics/Molecular Mechanics (QM/MM):
For studying specific reactive events or regions of high electronic density, QM/MM methods combine the accuracy of quantum mechanics for a small region of interest with the efficiency of classical mechanics for the rest of the system. This allows for a more accurate description of chemical reactions within proteins.
Challenges and Limitations of MD Simulations
Despite their power, MD simulations are not without limitations:
-
Computational Cost: Simulating large systems over long timescales remains computationally demanding, limiting the size and duration of simulations.
-
Force Field Accuracy: The accuracy of MD simulations is inherently dependent on the accuracy of the force field used. While force fields are constantly being improved, they are still approximations of reality, and inaccuracies can lead to artifacts in the simulation results.
-
Sampling Limitations: Even with enhanced sampling techniques, accessing the entire conformational space of a protein can be challenging, particularly for large and complex systems.
-
Solvent Effects: Explicitly including solvent molecules in simulations significantly increases the computational cost. However, the solvent plays a crucial role in protein structure and dynamics, and its omission can lead to inaccurate results.
The Future of MD Simulations in Protein Structure Research
MD simulations are constantly evolving, with ongoing advancements in computational hardware, algorithms, and force fields. The development of more accurate force fields, incorporating explicit polarization effects and improved descriptions of long-range interactions, will enhance the predictive power of simulations. The integration of machine learning techniques promises to accelerate data analysis and improve the sampling efficiency of simulations. As computational power continues to increase, we can anticipate even longer and more accurate simulations, providing an increasingly detailed and comprehensive picture of the dynamic nature of protein structures.
Conclusion: A Dynamic View of Protein Structure
Molecular dynamics simulations are indispensable tools for studying protein structure and dynamics. They offer a powerful way to go beyond static structural snapshots, revealing the intricate movements and fluctuations that are essential for protein function. While challenges remain, the continued development and application of MD simulations promise to provide increasingly refined and detailed insights into the dynamic world of proteins, leading to significant advancements in various scientific fields. The combined power of experimental techniques and computational simulations forms a crucial synergy in our ongoing quest to understand the fundamental mechanisms of life at the molecular level.
Latest Posts
Latest Posts
-
Construction And Building Materials Impact Factor
May 28, 2025
-
What Material Is Transported In The Sinusoids Of The Liver
May 28, 2025
-
Mom Has Sex With Daughter And Son
May 28, 2025
-
One Area In Which Aggregate Planning Decisions Are Made Is
May 28, 2025
-
What Does The Lateral Hypothalamus Do
May 28, 2025
Related Post
Thank you for visiting our website which covers about Molecular Dynamics Simulations Show That The Structure Of Proteins . We hope the information provided has been useful to you. Feel free to contact us if you have any questions or need further assistance. See you next time and don't miss to bookmark.