Maximum Number Of Electrons In 3d Sublevel
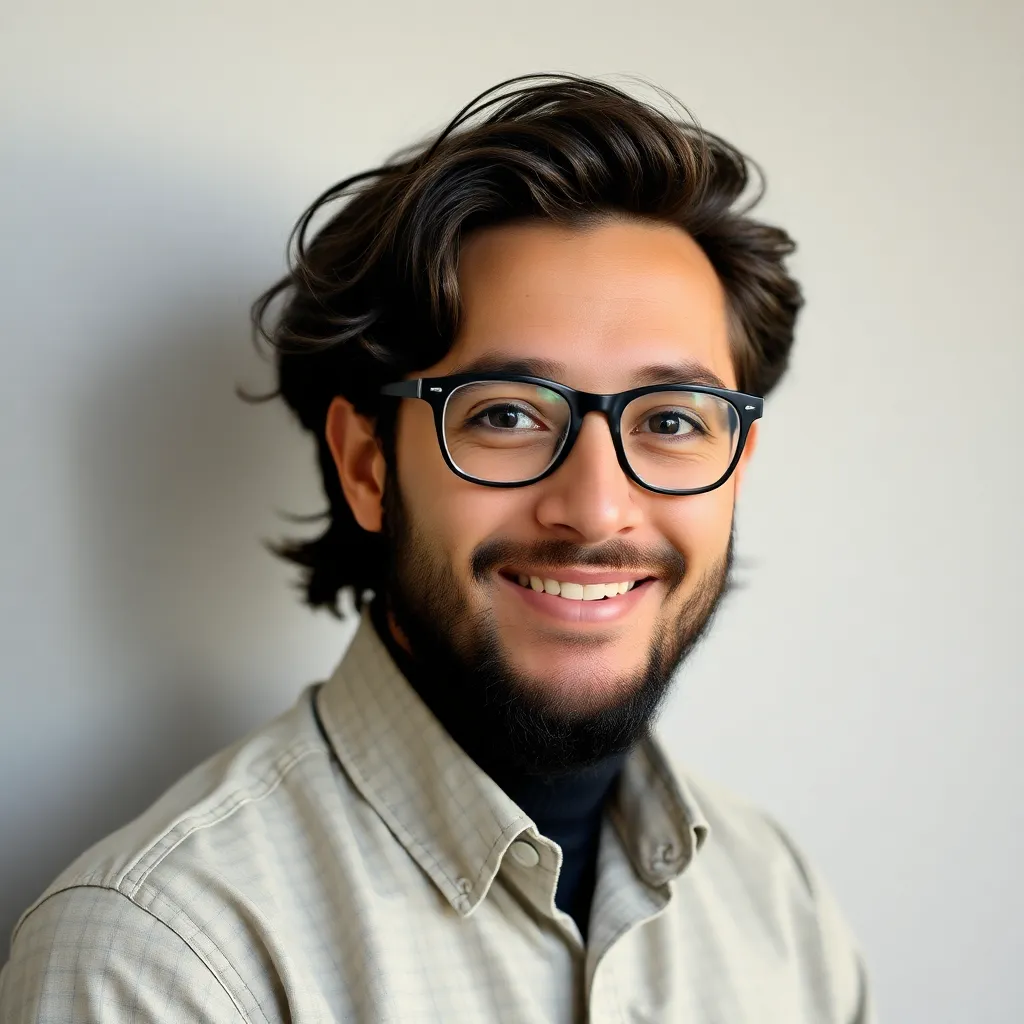
listenit
Apr 13, 2025 · 5 min read

Table of Contents
Maximum Number of Electrons in the 3d Sublevel: A Deep Dive into Atomic Structure
Understanding the maximum number of electrons a subshell can hold is fundamental to grasping the principles of atomic structure and chemical behavior. This article delves into the intricacies of electron configuration, focusing specifically on the 3d subshell and its capacity for electrons. We'll explore the quantum numbers, the Pauli Exclusion Principle, Hund's Rule, and the implications of this electron capacity on the properties of transition metals.
Understanding Electron Configuration
Before we dive into the specifics of the 3d subshell, let's lay the groundwork by reviewing electron configuration. Electron configuration describes the arrangement of electrons within an atom's energy levels and sublevels. This arrangement dictates an atom's chemical properties and its behavior in various reactions.
The arrangement follows specific rules:
-
Principal Quantum Number (n): This number defines the main energy level or shell. It can be any positive integer (1, 2, 3, and so on). Higher values of n correspond to higher energy levels and greater distance from the nucleus.
-
Azimuthal Quantum Number (l): This number defines the subshell within a principal energy level. It can have integer values ranging from 0 to n - 1. The subshells are designated by letters: l = 0 (s), l = 1 (p), l = 2 (d), l = 3 (f), and so on.
-
Magnetic Quantum Number (ml): This number describes the orientation of the orbital within a subshell. It can have integer values ranging from -l to +l, including 0. For example, a p subshell (l = 1) has three orbitals (ml = -1, 0, +1).
-
Spin Quantum Number (ms): This quantum number describes the intrinsic angular momentum of an electron, often visualized as spin "up" (+1/2) or spin "down" (-1/2).
These four quantum numbers uniquely identify each electron within an atom. No two electrons can have the identical set of four quantum numbers, a principle known as the Pauli Exclusion Principle.
The 3d Subshell: Orbitals and Electron Capacity
Now, let's focus on the 3d subshell. The principal quantum number (n) is 3, indicating it resides in the third main energy level. The azimuthal quantum number (l) is 2, which designates it as a d subshell.
The magnetic quantum number (ml) for the 3d subshell can have values from -2 to +2, meaning there are five 3d orbitals:
- ml = -2
- ml = -1
- ml = 0
- ml = +1
- ml = +2
Each of these five 3d orbitals can hold a maximum of two electrons, one with spin up (+1/2) and one with spin down (-1/2), according to the Pauli Exclusion Principle.
Calculating the Maximum Number of Electrons in 3d
Therefore, the maximum number of electrons the 3d subshell can accommodate is calculated as follows:
Number of orbitals x electrons per orbital = Total number of electrons
5 orbitals x 2 electrons/orbital = 10 electrons
The 3d subshell can hold a maximum of 10 electrons.
Hund's Rule and Electron Filling
While the maximum capacity is 10 electrons, the order in which electrons fill the 3d orbitals follows Hund's Rule. Hund's Rule states that electrons will individually occupy each orbital within a subshell before doubling up in any one orbital. This is because electrons repel each other, and it's energetically more favorable for them to occupy separate orbitals with parallel spins initially. Only after each orbital has one electron will electrons start pairing up within orbitals.
Implications for Transition Metals
The 3d subshell plays a crucial role in the properties of transition metals. Transition metals are elements whose atoms have incompletely filled d subshells. The presence of these partially filled 3d orbitals is responsible for many of the characteristic properties of transition metals, including:
-
Variable Oxidation States: The ability of transition metals to exist in multiple oxidation states is due to the relatively small energy differences between the 3d and 4s orbitals. Electrons can be easily removed from both, leading to different oxidation states.
-
Formation of Colored Compounds: The d electrons in transition metals can absorb specific wavelengths of light, leading to the characteristic colors of their compounds. This is due to electronic transitions between different d orbitals.
-
Catalytic Activity: Many transition metals and their compounds act as catalysts due to their ability to readily accept or donate electrons. The presence of partially filled 3d orbitals allows them to facilitate chemical reactions by forming intermediate complexes.
-
Magnetic Properties: The presence of unpaired electrons in the 3d orbitals leads to paramagnetism, where the substance is weakly attracted to a magnetic field. Some transition metal compounds can exhibit ferromagnetism or antiferromagnetism, depending on the arrangement of unpaired electrons.
Exceptions and Irregularities
While the general rules of electron configuration are quite reliable, there are some exceptions, particularly within the transition metals. These exceptions can sometimes arise due to the energy differences between the 3d and 4s orbitals becoming comparable or due to stability gained from completely filled or half-filled subshells. These subtle energy variations can influence the order in which orbitals are filled, leading to slightly different electron configurations than predicted by simple rules. Careful consideration of these exceptions is often needed to understand the properties of certain transition metal compounds.
Advanced Concepts and Further Exploration
The study of electron configuration and atomic structure extends far beyond the basics covered here. More advanced concepts include:
-
Relativistic effects: At higher atomic numbers, relativistic effects become increasingly significant, influencing the energy levels and electron configurations of elements.
-
Quantum mechanical calculations: Sophisticated quantum mechanical calculations provide more accurate predictions of electron configurations, taking into account electron-electron interactions and other complexities.
-
Spectroscopy: Spectroscopic techniques, such as UV-Vis spectroscopy and X-ray photoelectron spectroscopy, provide experimental data that can be used to verify and refine theoretical models of electron configuration.
Conclusion
The 3d subshell, with its capacity to hold a maximum of 10 electrons, is a critical component in understanding the structure and behavior of atoms, particularly transition metals. The principles of quantum numbers, the Pauli Exclusion Principle, and Hund's Rule are essential to accurately determine and interpret electron configurations. Understanding these concepts is fundamental to various aspects of chemistry, from predicting chemical reactions to explaining the diverse properties of the elements. Further exploration of advanced concepts can lead to a deeper understanding of the intricacies of atomic structure and the fascinating world of quantum mechanics.
Latest Posts
Latest Posts
-
R 2 X 2 Y 2
Apr 14, 2025
-
Can Rate Of Change Be Negative
Apr 14, 2025
-
Which Chemical Change Will Convert Adp To Atp
Apr 14, 2025
-
What Is The Value Of Eight
Apr 14, 2025
-
Finding The Radian Measure Of The Central Angle
Apr 14, 2025
Related Post
Thank you for visiting our website which covers about Maximum Number Of Electrons In 3d Sublevel . We hope the information provided has been useful to you. Feel free to contact us if you have any questions or need further assistance. See you next time and don't miss to bookmark.