How To Know If A Molecule Is Planar
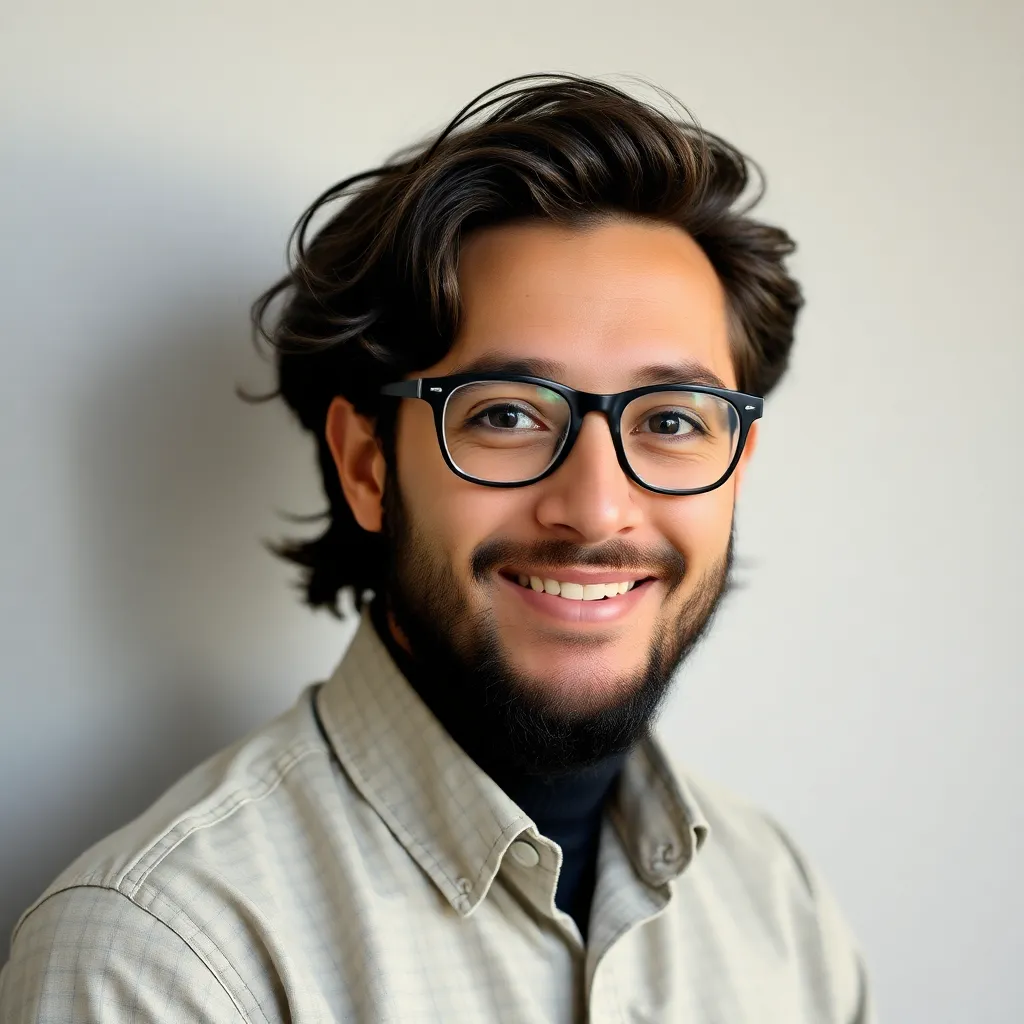
listenit
May 12, 2025 · 7 min read
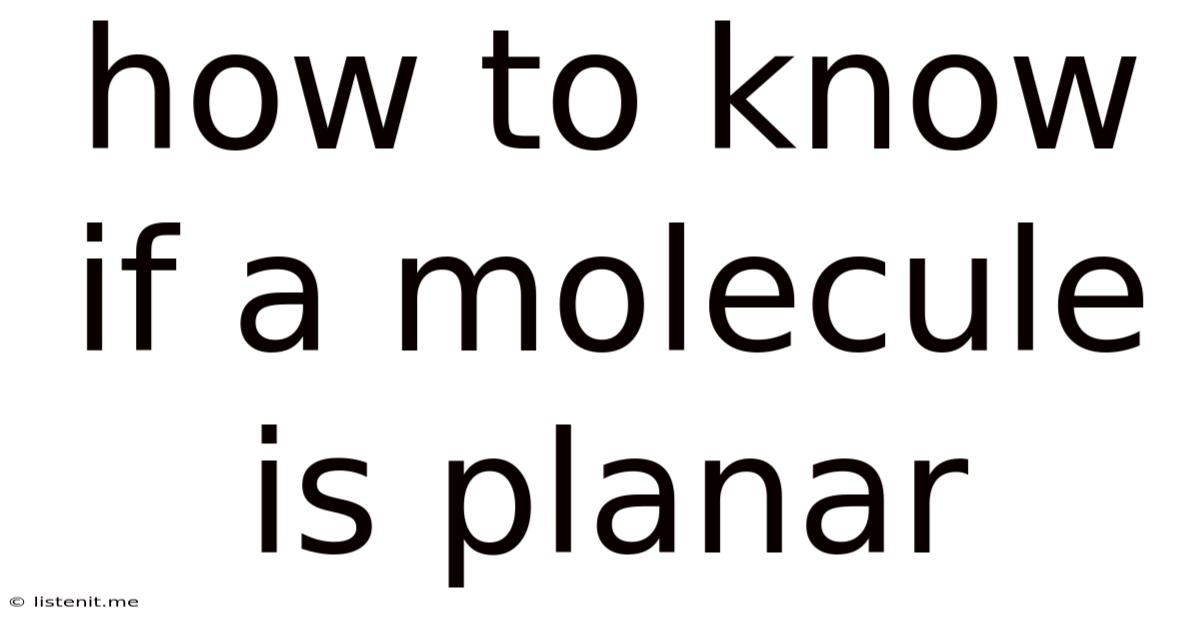
Table of Contents
How to Know if a Molecule is Planar: A Comprehensive Guide
Determining whether a molecule is planar is crucial in various fields, including chemistry, biochemistry, and materials science. Planarity significantly influences a molecule's properties, such as reactivity, absorption of light, and overall stability. This comprehensive guide delves into various methods and concepts to help you confidently assess the planarity of a molecule.
Understanding Molecular Geometry and Planarity
Before diving into the specifics, let's establish a clear understanding of fundamental concepts. Molecular geometry describes the three-dimensional arrangement of atoms in a molecule. A planar molecule is one where all its atoms lie in the same plane. This means you could draw the molecule on a flat piece of paper without distorting any bond angles. Conversely, a non-planar molecule has atoms that are positioned above and below a reference plane.
Several factors determine a molecule's planarity, including:
- Hybridization: The type of orbital hybridization significantly impacts the molecular geometry. For example, sp<sup>2</sup> hybridized atoms typically lead to planar structures.
- Lone pairs of electrons: Lone pairs occupy space and often distort molecular geometry, potentially disrupting planarity.
- Bonding: Multiple bonds, especially double and triple bonds, constrain the atoms' movement and can enforce planarity.
- Steric hindrance: Bulky substituents can cause steric repulsion, forcing atoms out of a plane.
Methods to Determine Molecular Planarity
Several methods can be employed to assess whether a molecule is planar. These methods range from simple visual inspection to sophisticated computational techniques.
1. Visual Inspection and VSEPR Theory
For simpler molecules, visual inspection combined with Valence Shell Electron Pair Repulsion (VSEPR) theory can often be sufficient. VSEPR predicts the geometry of molecules based on the repulsion between electron pairs (both bonding and lone pairs) around a central atom.
How to use VSEPR:
- Draw the Lewis structure: Determine the number of valence electrons for each atom and arrange them to satisfy the octet rule (or duet rule for hydrogen).
- Count electron domains: An electron domain is a region of electron density, encompassing bonding pairs and lone pairs.
- Predict the electron domain geometry: This geometry is determined by the number of electron domains:
- 2 domains: linear
- 3 domains: trigonal planar
- 4 domains: tetrahedral
- 5 domains: trigonal bipyramidal
- 6 domains: octahedral
- Determine the molecular geometry: This considers the positions of only the atoms, ignoring lone pairs. Lone pairs affect the bond angles and overall shape but may not always prevent planarity.
Example: BF<sub>3</sub> (Boron trifluoride). Boron has three valence electrons, and each fluorine contributes one, giving a total of six. This leads to three electron domains (three bonding pairs) around boron. The electron domain geometry and molecular geometry are both trigonal planar, making BF<sub>3</sub> a planar molecule.
Limitations: VSEPR is a simplified model and doesn't always accurately predict the geometry of complex molecules with multiple central atoms and significant steric effects.
2. Hybridization and Molecular Orbital Theory
Hybridization describes the mixing of atomic orbitals to form hybrid orbitals with different shapes and energies. The type of hybridization is a strong indicator of molecular geometry.
- sp<sup>2</sup> hybridization: This hybridization results in three sp<sup>2</sup> hybrid orbitals in a trigonal planar arrangement and one unhybridized p orbital. Molecules with sp<sup>2</sup> hybridized central atoms are typically planar, provided other factors don't disrupt this geometry. Examples include benzene (C<sub>6</sub>H<sub>6</sub>) and formaldehyde (CH<sub>2</sub>O).
- sp<sup>3</sup> hybridization: This hybridization leads to four sp<sup>3</sup> hybrid orbitals in a tetrahedral arrangement. Molecules with sp<sup>3</sup> hybridized atoms are generally non-planar. However, there are exceptions. For instance, cyclohexane can adopt a planar conformation, though it's less stable than its chair conformation.
- sp hybridization: This results in a linear geometry. Molecules with sp hybridized central atoms are linear and, therefore, planar.
Molecular orbital theory provides a more advanced and accurate description of bonding, allowing for a more precise prediction of molecular geometry. However, it requires a deeper understanding of quantum mechanics.
3. Analyzing Bond Angles
In planar molecules, the bond angles around each atom will often adhere to ideal values predicted by VSEPR theory (e.g., 120° for trigonal planar geometry, 180° for linear geometry). Deviations from these ideal angles can suggest a non-planar structure. X-ray crystallography and other spectroscopic techniques can provide precise measurements of bond angles.
4. Spectroscopic Techniques
Various spectroscopic techniques can provide valuable information about molecular structure, indirectly indicating planarity. Infrared (IR) spectroscopy can reveal the presence of functional groups and their vibrational modes, which can be consistent with a planar or non-planar structure. Nuclear Magnetic Resonance (NMR) spectroscopy can provide insights into the spatial arrangement of atoms, particularly through coupling constants and chemical shifts. Raman spectroscopy, similar to IR, provides vibrational information that can be used to infer molecular geometry.
5. Computational Chemistry
For complex molecules, computational chemistry methods, such as density functional theory (DFT) and ab initio calculations, provide highly accurate predictions of molecular geometry, including planarity. These methods use sophisticated algorithms to solve the Schrödinger equation, providing detailed information about the molecule's electronic structure and geometry. Software packages like Gaussian, GAMESS, and ORCA are widely used for these computations. The results usually include optimized structures, providing accurate bond lengths, angles, and dihedral angles, which can definitively determine planarity.
Factors that Influence Planarity and Non-planarity
Several factors beyond hybridization and VSEPR can influence a molecule’s planarity:
1. Conjugation and Resonance
Molecules with extensive conjugation systems, where alternating single and multiple bonds allow for electron delocalization, often prefer planar conformations. This is because planarity allows for maximum overlap of p-orbitals, leading to enhanced stability through resonance. Examples include benzene, naphthalene, and many aromatic compounds.
2. Steric Effects
Steric hindrance between bulky substituents can force a molecule out of planarity. The repulsive forces between these groups can overcome the energetic advantage of planarity. Consider cyclohexane: while it could theoretically adopt a planar structure, the significant steric interactions between hydrogens would make it incredibly unstable. The chair conformation, which is non-planar, is significantly more stable due to reduced steric hindrance.
3. Lone Pairs of Electrons
Lone pairs of electrons on a central atom can significantly affect molecular geometry. They occupy space and repel bonding pairs, often causing distortions from ideal geometries and resulting in non-planar structures. Ammonia (NH<sub>3</sub>), for instance, has a pyramidal shape due to the lone pair on the nitrogen atom, preventing it from being planar.
4. Ring Strain
In cyclic molecules, ring strain can influence planarity. Small rings, like cyclopropane, are highly strained and non-planar. Larger rings are often more flexible and can adopt various conformations, including planar ones. However, larger rings often prefer non-planar conformations to minimize steric hindrance.
Examples: Planar vs. Non-planar Molecules
Let's examine some concrete examples:
Planar Molecules:
- Benzene (C<sub>6</sub>H<sub>6</sub>): The classic example of a planar molecule due to its sp<sup>2</sup> hybridized carbons and delocalized pi electrons.
- Formaldehyde (CH<sub>2</sub>O): The central carbon is sp<sup>2</sup> hybridized.
- Boron trifluoride (BF<sub>3</sub>): The central boron is sp<sup>2</sup> hybridized.
- Carbon dioxide (CO<sub>2</sub>): Linear molecule with sp hybridized carbon.
Non-planar Molecules:
- Ammonia (NH<sub>3</sub>): The lone pair on nitrogen distorts the tetrahedral geometry.
- Methane (CH<sub>4</sub>): The central carbon is sp<sup>3</sup> hybridized, resulting in a tetrahedral shape.
- Water (H<sub>2</sub>O): The lone pairs on oxygen cause a bent geometry.
- Cyclohexane (C<sub>6</sub>H<sub>12</sub>): Prefers a chair conformation to minimize steric hindrance.
Conclusion
Determining the planarity of a molecule requires a multi-faceted approach. Combining VSEPR theory, hybridization analysis, spectroscopic techniques, and computational chemistry allows for accurate determination, especially for complex molecules. Understanding the interplay of factors such as conjugation, steric effects, lone pairs, and ring strain is crucial for a comprehensive understanding of molecular geometry and planarity. This knowledge is essential for predicting and understanding various molecular properties and their applications in various scientific disciplines.
Latest Posts
Related Post
Thank you for visiting our website which covers about How To Know If A Molecule Is Planar . We hope the information provided has been useful to you. Feel free to contact us if you have any questions or need further assistance. See you next time and don't miss to bookmark.