How To Go From Alkane To Alkene
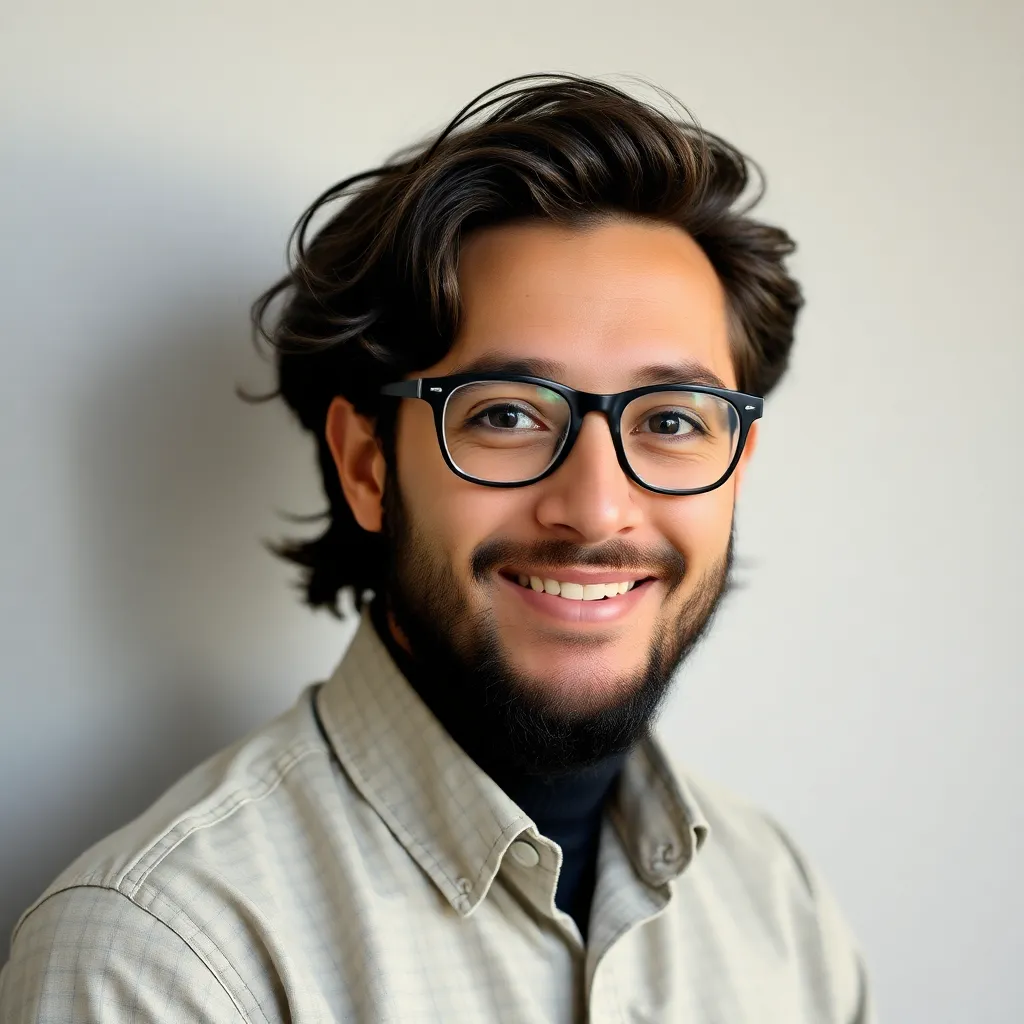
listenit
Apr 16, 2025 · 6 min read

Table of Contents
How to Go From Alkane to Alkene: A Comprehensive Guide
Alkanes and alkenes are fundamental classes of hydrocarbons, differing significantly in their structure and reactivity. Alkanes, saturated hydrocarbons, contain only single bonds between carbon atoms, while alkenes, unsaturated hydrocarbons, possess at least one carbon-carbon double bond. This double bond introduces a region of higher electron density and significantly alters the chemical behavior of the molecule. Transforming an alkane into an alkene, therefore, requires a chemical process that introduces this unsaturation. This process, broadly termed dehydrogenation, involves the removal of hydrogen atoms from adjacent carbon atoms, forming the characteristic double bond. This article will delve into various methods for achieving this transformation, discussing their mechanisms, applications, and limitations.
Understanding the Fundamentals: Alkanes vs. Alkenes
Before exploring the conversion methods, it's crucial to understand the key distinctions between alkanes and alkenes:
Alkanes: The Saturated Hydrocarbons
- Structure: Characterized by single carbon-carbon bonds (C-C) and carbon-hydrogen bonds (C-H). They exhibit a tetrahedral geometry around each carbon atom.
- Reactivity: Relatively inert due to the strong C-C and C-H bonds. They primarily undergo combustion and free radical substitution reactions.
- Examples: Methane (CH₄), Ethane (C₂H₆), Propane (C₃H₈), Butane (C₄H₁₀)
Alkenes: The Unsaturated Hydrocarbons
- Structure: Contains at least one carbon-carbon double bond (C=C). This double bond consists of one sigma (σ) bond and one pi (π) bond. The geometry around the double bond is planar.
- Reactivity: Significantly more reactive than alkanes due to the presence of the π-bond, which is a region of high electron density susceptible to electrophilic attack. They readily undergo addition reactions.
- Examples: Ethene (C₂H₄), Propene (C₃H₆), But-1-ene (C₄H₈)
Methods for Converting Alkanes to Alkenes: Dehydrogenation Reactions
Several methods can facilitate the conversion of alkanes to alkenes. These methods generally involve dehydrogenation, a process that removes hydrogen atoms from adjacent carbon atoms, creating a double bond. The specific method chosen often depends on the desired alkene product, the nature of the alkane starting material, and other practical considerations.
1. Catalytic Dehydrogenation:
This is a widely used industrial method for alkene production, particularly for the conversion of ethane to ethene and propane to propene. It involves passing the alkane over a heated catalyst, typically a transition metal catalyst like chromium oxide (Cr₂O₃) or platinum (Pt).
- Mechanism: The catalyst facilitates the removal of hydrogen molecules (H₂) from the alkane, leaving behind a double bond. The reaction is typically endothermic, requiring heat input to drive the equilibrium towards alkene formation.
- Advantages: Relatively simple process, high yields achievable under optimized conditions.
- Disadvantages: High temperatures are required, leading to potential side reactions and catalyst deactivation. Selectivity can be a challenge, especially for larger alkanes.
2. Thermal Cracking (Pyrolysis):
This method involves heating the alkane to high temperatures in the absence of a catalyst. The high temperature provides the energy needed to break C-H and C-C bonds, leading to fragmentation and the formation of alkenes amongst other products.
- Mechanism: The high temperature promotes homolytic cleavage of C-C and C-H bonds, generating highly reactive free radicals. These radicals undergo various reactions, including β-scission (breaking of a bond adjacent to a radical center), resulting in alkene formation.
- Advantages: Relatively simple setup, can handle a wide range of alkanes.
- Disadvantages: Low selectivity, producing a mixture of products, often requiring further separation steps. High energy consumption.
3. Free Radical Halogenation Followed by Dehydrohalogenation:
This two-step process involves first introducing halogen atoms (chlorine or bromine) to the alkane through free radical halogenation, followed by dehydrohalogenation to eliminate the halogen and hydrogen, creating a double bond.
- Step 1: Free Radical Halogenation: Initiated by UV light, this step replaces a hydrogen atom with a halogen atom, forming a haloalkane. The reaction is not very selective, leading to a mixture of isomers.
- Step 2: Dehydrohalogenation: Treatment of the haloalkane with a strong base (like alcoholic KOH) removes the halogen and a hydrogen atom from adjacent carbon atoms, forming an alkene. This step follows Zaitsev's rule, favoring the formation of the more substituted alkene (the one with more alkyl groups attached to the double bond).
- Advantages: Can be used for a variety of alkanes, offering some control over the position of the double bond through the choice of base and reaction conditions.
- Disadvantages: Two-step process, lower overall yield due to the cumulative yield losses in each step, formation of multiple isomers.
4. Oxidation Followed by Reduction (Indirect Method):
This less common method involves first oxidizing the alkane to an alcohol, followed by a dehydration reaction to eliminate water and form an alkene.
- Step 1: Oxidation: Typically requires strong oxidizing agents to convert the alkane to an alcohol. This step is often challenging and not very efficient for alkanes.
- Step 2: Dehydration: The alcohol is then dehydrated using an acid catalyst (like sulfuric acid or phosphoric acid) to form an alkene. This step follows Zaitsev's rule.
- Advantages: Can be selectively used for specific positions.
- Disadvantages: Often less efficient than direct dehydrogenation, requiring multiple steps and harsh conditions.
Choosing the Right Method: Factors to Consider
The optimal method for converting an alkane to an alkene depends on several factors:
- Type of Alkane: Simple alkanes like ethane and propane are best suited for catalytic dehydrogenation. Larger, more complex alkanes may require thermal cracking or free radical halogenation followed by dehydrohalogenation.
- Desired Alkene Product: If a specific alkene isomer is desired, free radical halogenation followed by dehydrohalogenation might offer more control, albeit with lower selectivity.
- Scale of Production: Catalytic dehydrogenation is preferred for large-scale industrial production due to its efficiency.
- Cost and Availability of Reagents and Catalysts: The choice of method will also depend on the cost and availability of the necessary reagents and catalysts.
Applications of Alkene Production from Alkanes
The conversion of alkanes to alkenes is crucial for various industrial applications:
- Polymer Production: Alkenes, especially ethene and propene, are the building blocks for a vast array of polymers, including polyethylene, polypropylene, and many other plastics.
- Petrochemical Industry: Alkenes serve as important intermediates in the synthesis of numerous chemicals, including solvents, detergents, and other valuable products.
- Fuel Production: Some alkenes are used directly as fuels or as components in gasoline blends.
Conclusion:
Converting alkanes to alkenes, fundamentally a dehydrogenation process, is a significant transformation in organic chemistry with far-reaching industrial applications. Various methods, each with its advantages and limitations, exist for achieving this conversion. The choice of method depends on factors such as the type of alkane, the desired alkene product, the scale of production, and economic considerations. Understanding these methods and their underlying mechanisms is critical for anyone involved in organic chemistry, petrochemical engineering, or related fields. Further research into developing more efficient, selective, and environmentally friendly methods for alkene production from alkanes remains an active area of investigation. The continuous optimization of these processes is crucial for meeting the growing global demand for alkenes and their derivative products.
Latest Posts
Latest Posts
-
Number Of Protons Neutrons And Electrons In Phosphorus
Apr 19, 2025
-
What Phase Do Homologous Chromosomes Separate
Apr 19, 2025
-
What Is The Gcf Of 60
Apr 19, 2025
-
Which Element Is The Most Reactive Metal
Apr 19, 2025
-
Which Of These Is An Example Of A Physical Property
Apr 19, 2025
Related Post
Thank you for visiting our website which covers about How To Go From Alkane To Alkene . We hope the information provided has been useful to you. Feel free to contact us if you have any questions or need further assistance. See you next time and don't miss to bookmark.