How To Find Rate Determining Step
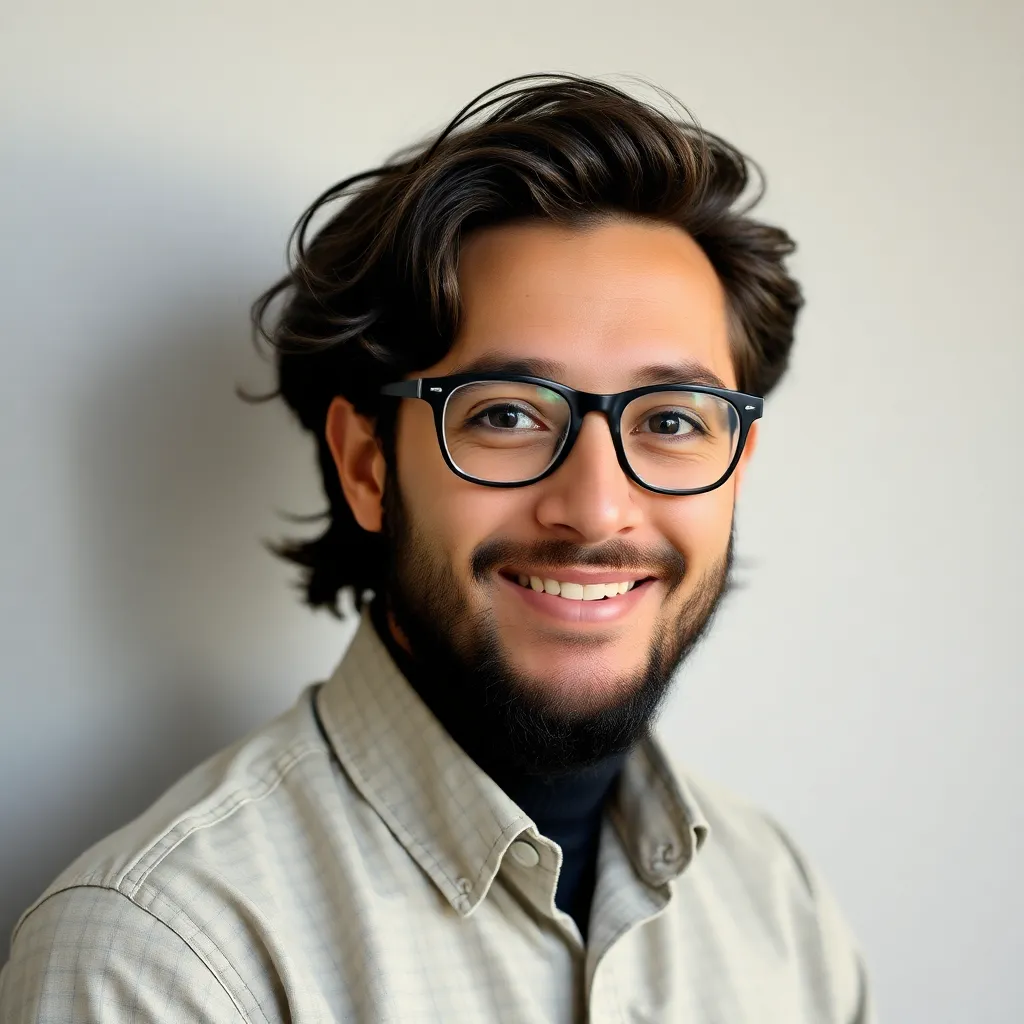
listenit
Apr 24, 2025 · 6 min read

Table of Contents
How to Find the Rate-Determining Step: A Comprehensive Guide
Understanding reaction mechanisms is crucial in chemistry, and a key aspect of this understanding lies in identifying the rate-determining step (RDS), also known as the rate-limiting step. This step dictates the overall speed of the reaction, regardless of how fast or slow other steps might be. This article will provide a comprehensive guide on how to find the rate-determining step, covering various approaches and scenarios.
What is the Rate-Determining Step?
Before delving into the methods of identification, let's solidify our understanding of the RDS. In a multi-step reaction mechanism, each step involves its own elementary reaction with a specific rate constant. The rate-determining step is the slowest elementary reaction in the entire mechanism. Its rate directly determines the overall rate of the reaction. Think of it like a bottleneck in a production line; even if other stages are highly efficient, the slowest stage will limit the overall output.
Methods for Identifying the Rate-Determining Step
Identifying the RDS isn't always straightforward and often requires a combination of experimental data and theoretical understanding of the reaction mechanism. Here are some common approaches:
1. Experimental Determination using Initial Rates
This is a powerful experimental technique. By systematically changing the initial concentrations of reactants and observing the effect on the initial rate of the reaction, we can deduce the rate law. The order of the reaction with respect to each reactant, as determined from the rate law, provides crucial clues about the RDS.
Example:
Consider a reaction with the following proposed mechanism:
- Step 1 (fast equilibrium): A + B <=> C
- Step 2 (slow): C + D -> E
If the rate law experimentally determined is Rate = k[A][B][D], this suggests that Step 2 is the RDS. The concentration of C, an intermediate, is incorporated into the rate law using the equilibrium expression from Step 1.
2. Analyzing the Reaction Mechanism
If a plausible reaction mechanism is already known or proposed, analyzing the individual steps can help identify the RDS. This involves considering:
- The slowest step: The step with the highest activation energy (Ea) or the smallest rate constant (k) is likely the RDS. Activation energy is a measure of the energy barrier that must be overcome for a reaction to occur. A higher activation energy implies a slower reaction rate.
- Intermediate concentrations: The rate law should only involve reactants and products, not intermediate species. If an intermediate appears in the rate law, it must be expressed in terms of reactant concentrations using equilibrium expressions from faster preceding steps. The appearance of an intermediate's concentration in the experimentally determined rate law indicates that the step producing that intermediate is likely not the RDS.
3. Steady-State Approximation
For complex mechanisms, the steady-state approximation can be invaluable. This approximation assumes that the concentration of any intermediate remains relatively constant throughout the reaction. This simplification allows us to express the concentration of the intermediate in terms of reactant concentrations, ultimately leading to a simplified rate law that can be compared to experimental data. The steady-state approximation works best when the concentration of the intermediate is much lower than the concentrations of the reactants and products.
How to Apply the Steady-State Approximation:
- Identify intermediates: Pinpoint any species that are produced and consumed during the reaction but do not appear in the overall stoichiometry.
- Write rate expressions: Write the rate expressions for the formation and consumption of each intermediate.
- Set the net rate to zero: Assume that the rate of formation equals the rate of consumption for each intermediate (d[intermediate]/dt = 0).
- Solve for the intermediate concentration: Solve the resulting equations for the concentration of each intermediate in terms of reactant concentrations.
- Substitute into the rate law: Substitute the expressions for intermediate concentrations into the rate law for the overall reaction.
This process simplifies the rate law, making it easier to compare to experimental data and identify the RDS.
4. Kinetic Isotope Effects (KIE)
KIE involves replacing an atom in a reactant molecule with its heavier isotope (e.g., replacing hydrogen with deuterium). If the rate of the reaction changes significantly upon isotopic substitution, it indicates that the bond involving that atom is broken or formed in the RDS. A substantial change in rate usually suggests that the bond is broken or formed in the rate-determining step. The magnitude of the KIE can offer insights into the nature of the transition state.
5. Computational Chemistry
Advances in computational chemistry offer powerful tools to investigate reaction mechanisms and identify the RDS. Methods such as density functional theory (DFT) can be used to calculate activation energies for each step in a proposed mechanism. The step with the highest calculated activation energy is likely the RDS. This computational approach complements experimental methods and provides valuable theoretical insights.
Examples and Detailed Explanations
Let's examine some detailed examples to illustrate these concepts:
Example 1: SN1 Reaction
The SN1 (Substitution Nucleophilic Unimolecular) reaction is a two-step mechanism:
- Step 1 (slow): R-X -> R+ + X- (ionization)
- Step 2 (fast): R+ + Nu- -> R-Nu (nucleophilic attack)
The RDS is Step 1, the ionization step, because it involves breaking a bond and creating a high-energy carbocation intermediate. The rate law for an SN1 reaction is first-order: Rate = k[R-X], confirming that only the concentration of the substrate affects the overall rate.
Example 2: SN2 Reaction
The SN2 (Substitution Nucleophilic Bimolecular) reaction is a concerted one-step mechanism:
Nu- + R-X -> Nu-R + X-
There is no intermediate, and the rate law is second-order: Rate = k[Nu-][R-X]. This signifies that the reaction proceeds through a single step where both the nucleophile and substrate are involved simultaneously. Since it is a single-step reaction, that step is also the RDS.
Example 3: A More Complex Mechanism
Consider a hypothetical three-step mechanism:
- Step 1 (fast equilibrium): A + B <=> C
- Step 2 (slow): C + D -> E
- Step 3 (fast): E -> F
Here, Step 2 is the RDS because it is the slowest step. The overall rate law would depend on the concentrations of A, B, and D. The intermediate C would not appear in the final rate law because its concentration is expressed through the equilibrium constant of Step 1.
Common Pitfalls and Considerations
- Oversimplification: Assuming a simple mechanism when a more complex one is involved can lead to inaccurate identification of the RDS.
- Ignoring intermediates: Failing to account for intermediates in the rate law can lead to incorrect conclusions.
- Experimental limitations: Obtaining accurate experimental data is crucial. Errors in measurement can affect the determination of the rate law and the identification of the RDS.
- Multiple RDS: In some rare cases, there might be more than one rate-determining step, particularly if the rates of two steps are similar and are much slower than all other steps.
Conclusion
Identifying the rate-determining step is a crucial aspect of understanding reaction mechanisms. A combination of experimental techniques, theoretical analysis, and computational methods often provides the most comprehensive understanding. By carefully considering the rate law, reaction mechanism, and employing techniques such as the steady-state approximation and kinetic isotope effects, chemists can accurately pinpoint the RDS and gain valuable insights into the dynamics of chemical reactions. Remember that context is key; the methods used will depend heavily on the complexity of the reaction and the available experimental data. This comprehensive guide provides a solid foundation for tackling this important aspect of chemical kinetics.
Latest Posts
Latest Posts
-
Is Potassium Hydroxide Soluble In Water
Apr 24, 2025
-
Lcm Of 2 7 And 3
Apr 24, 2025
-
Elements In The Periodic Table Are Arranged According To Their
Apr 24, 2025
-
What Is The Middle Of An Atom Called
Apr 24, 2025
-
2 Or More Elements Chemically Combined
Apr 24, 2025
Related Post
Thank you for visiting our website which covers about How To Find Rate Determining Step . We hope the information provided has been useful to you. Feel free to contact us if you have any questions or need further assistance. See you next time and don't miss to bookmark.