How To Find Bond Dissociation Energy
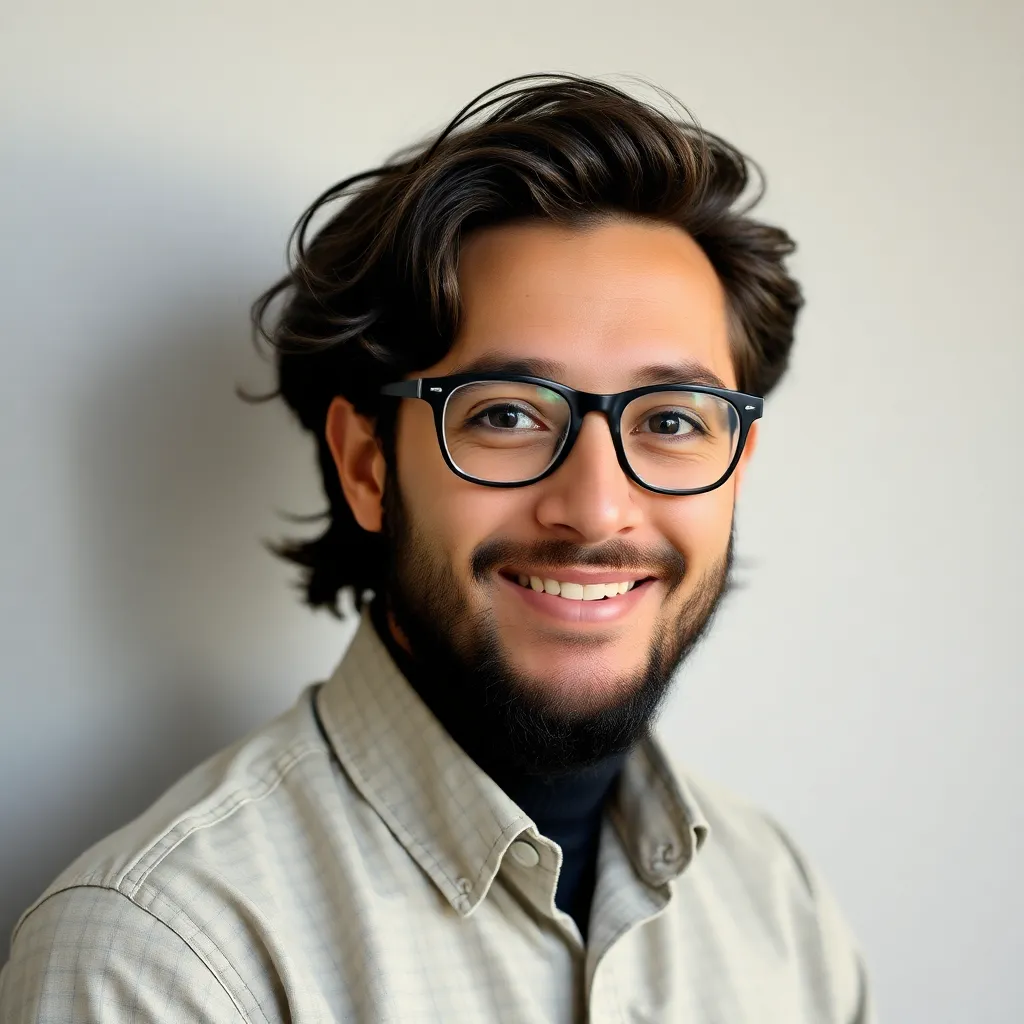
listenit
May 12, 2025 · 6 min read
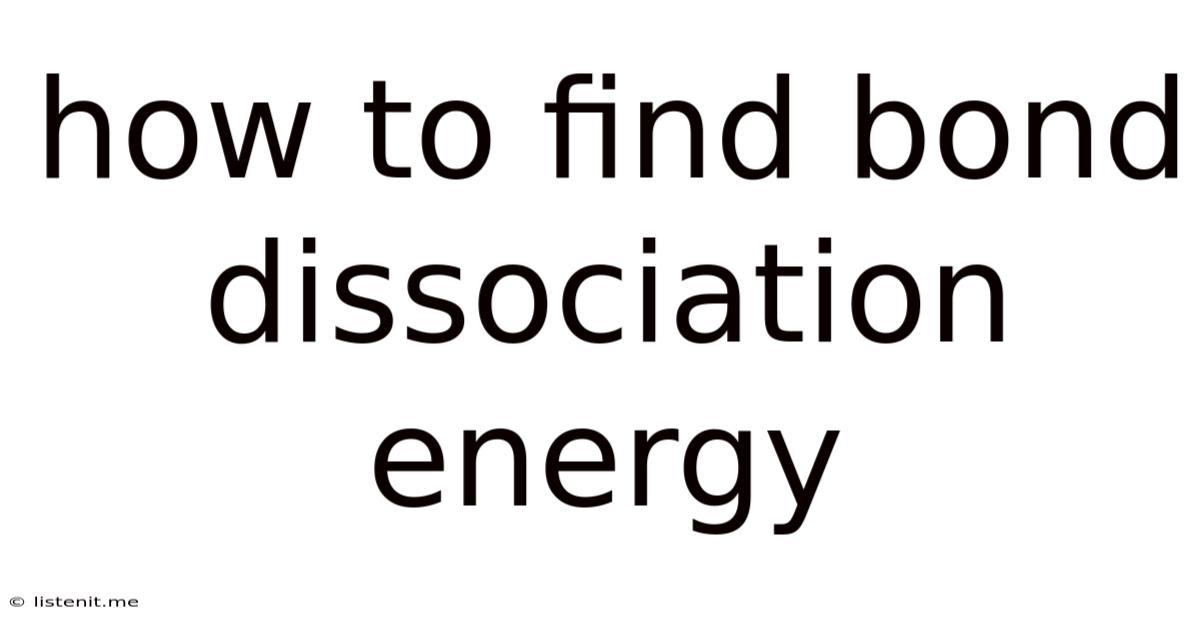
Table of Contents
How to Find Bond Dissociation Energy: A Comprehensive Guide
Bond dissociation energy (BDE), also known as bond strength, is a crucial concept in chemistry. It represents the amount of energy required to break one mole of a specific type of bond in a gaseous molecule. Understanding how to find BDE is vital for predicting reaction pathways, understanding reaction mechanisms, and estimating the stability of molecules. This comprehensive guide delves into various methods for determining bond dissociation energies, explaining the underlying principles and practical applications.
Understanding Bond Dissociation Energy (BDE)
Before exploring the methods, let's solidify our understanding of BDE. It's important to remember that BDE is always positive, signifying that energy is required to break a bond. The higher the BDE, the stronger the bond. This strength depends on factors such as the type of bond (single, double, triple), the atoms involved, and the molecular environment. For instance, a C-C single bond will have a different BDE than a C=C double bond or a C≡C triple bond. Similarly, the BDE of a C-H bond will vary depending on the other atoms bonded to the carbon atom.
Homolytic Cleavage vs. Heterolytic Cleavage
It's crucial to distinguish between homolytic and heterolytic cleavage when discussing bond dissociation. Homolytic cleavage involves the symmetrical breaking of a bond, with each atom retaining one electron from the shared pair. This is the type of bond breakage that BDE directly measures. In contrast, heterolytic cleavage involves the asymmetrical breaking of a bond, with one atom retaining both electrons from the shared pair, forming ions. BDE does not directly measure this type of bond breakage.
Methods for Determining Bond Dissociation Energies
Determining the BDE experimentally can be challenging and often requires sophisticated techniques. Several methods are employed, each with its advantages and limitations.
1. Experimental Determination using Calorimetry
Calorimetry is a direct method for measuring the heat absorbed or released during a chemical reaction. By carefully measuring the heat change associated with bond breaking, the BDE can be calculated. This approach typically involves reactions in the gas phase to ensure accurate measurements. However, this method can be quite complex and requires precise control of experimental conditions, including temperature and pressure.
Process: A known amount of the gaseous molecule is subjected to a controlled process that breaks the specific bond of interest. The heat absorbed (endothermic) during the bond breaking is measured using a calorimeter. This heat change, when appropriately converted, directly yields the BDE.
Limitations: This method can be challenging for weak bonds or bonds within complex molecules where multiple bond breakages might occur simultaneously. Accurate measurement of the heat absorbed is also crucial and requires precise instrumentation.
2. Spectroscopic Methods
Spectroscopic techniques, such as photoelectron spectroscopy (PES) and infrared (IR) spectroscopy, can provide valuable information about bond strengths. These methods analyze the energy of photons absorbed or emitted during molecular transitions, which can be related to the energy required to break specific bonds. PES, in particular, is quite effective in determining BDEs by analyzing the energy needed to remove an electron from a molecule, which often correlates with bond strength.
Process: Molecules are exposed to photons of varying energies. The absorption or emission of photons are measured, providing information about the energy levels within the molecule, including those associated with bond strengths. Analysis of the spectra allows for the determination of BDEs.
Limitations: The interpretation of spectroscopic data can be complex, requiring specialized knowledge and sophisticated software. The method may not be applicable to all types of molecules or bonds.
3. Computational Methods (Quantum Chemistry)
Computational chemistry offers powerful tools for predicting BDEs. Using sophisticated software packages and quantum mechanical calculations, researchers can model molecules and calculate the energy required to break specific bonds. Methods like Density Functional Theory (DFT) and coupled cluster (CC) calculations are commonly used for this purpose.
Process: A detailed computational model of the molecule is created. The energy of the molecule is calculated both with the intact bond and with the bond broken. The difference in energy corresponds to the BDE. The accuracy of the calculation depends on the level of theory and the basis set employed.
Limitations: While computational methods are increasingly accurate, they are still subject to approximations and limitations. The computational cost can be high for large molecules or complex systems. Accurate prediction requires careful selection of computational methods and basis sets. Validation against experimental data is always crucial.
4. Thermochemical Cycles
Thermochemical cycles utilize Hess's Law, which states that the enthalpy change for a reaction is independent of the pathway taken. By combining known enthalpy changes for various reactions that involve the bond of interest, one can indirectly determine the BDE. This approach is particularly useful when direct experimental measurement is difficult or impossible.
Process: A series of reactions is designed where the bond breakage of interest is a component of the overall reaction. The enthalpy changes for the individual reactions are either measured experimentally or obtained from literature. Using Hess's Law, the enthalpy change for the bond dissociation reaction can be calculated, giving the BDE.
Limitations: The accuracy of the BDE depends on the accuracy of the enthalpy changes used in the cycle. The method is less direct than other approaches and may require a considerable amount of thermochemical data.
Factors Affecting Bond Dissociation Energy
Several factors influence the BDE of a bond:
- Bond Order: Higher bond orders (double, triple bonds) generally lead to higher BDEs due to increased electron density and stronger attractive forces between atoms.
- Electronegativity: The difference in electronegativity between the bonded atoms can affect the BDE. A larger electronegativity difference can lead to a more polar bond, which can sometimes be weaker, leading to a lower BDE. However, this relationship isn't always straightforward.
- Hybridization: The hybridization of the atoms involved in the bond influences the BDE. For example, sp-hybridized carbon atoms form stronger bonds than sp<sup>3</sup>-hybridized carbon atoms.
- Steric Effects: Bulky groups surrounding the bond can cause steric hindrance, reducing the overlap between atomic orbitals and weakening the bond, leading to a lower BDE.
- Resonance: The presence of resonance structures can stabilize a molecule, increasing the BDE.
Applications of Bond Dissociation Energy
Understanding BDEs is essential across various chemical disciplines:
- Reaction Kinetics: BDE is crucial for predicting reaction rates and mechanisms. Reactions involving weaker bonds are often faster than those involving stronger bonds.
- Thermochemistry: BDE is a fundamental parameter in thermochemical calculations, enabling the prediction of reaction enthalpies and equilibrium constants.
- Combustion: Understanding BDEs is vital in studying combustion processes, particularly in predicting the energy released during fuel combustion.
- Material Science: BDEs help in designing and characterizing new materials with specific properties, such as strength and stability.
- Drug Design: BDEs are useful in predicting the stability and reactivity of drug molecules, influencing drug design and development.
Conclusion
Determining bond dissociation energy is a crucial aspect of chemistry, with significant implications across many fields. While experimental methods provide direct measurements, they can be challenging and may not be suitable for all situations. Computational methods offer valuable alternatives, providing increasingly accurate predictions of BDEs. The choice of method depends on factors such as the complexity of the molecule, the availability of resources, and the desired level of accuracy. A thorough understanding of BDE and the various methods for its determination is essential for anyone working in chemistry or related fields.
Latest Posts
Related Post
Thank you for visiting our website which covers about How To Find Bond Dissociation Energy . We hope the information provided has been useful to you. Feel free to contact us if you have any questions or need further assistance. See you next time and don't miss to bookmark.