How Many Valence Electrons Does Transition Metals Have
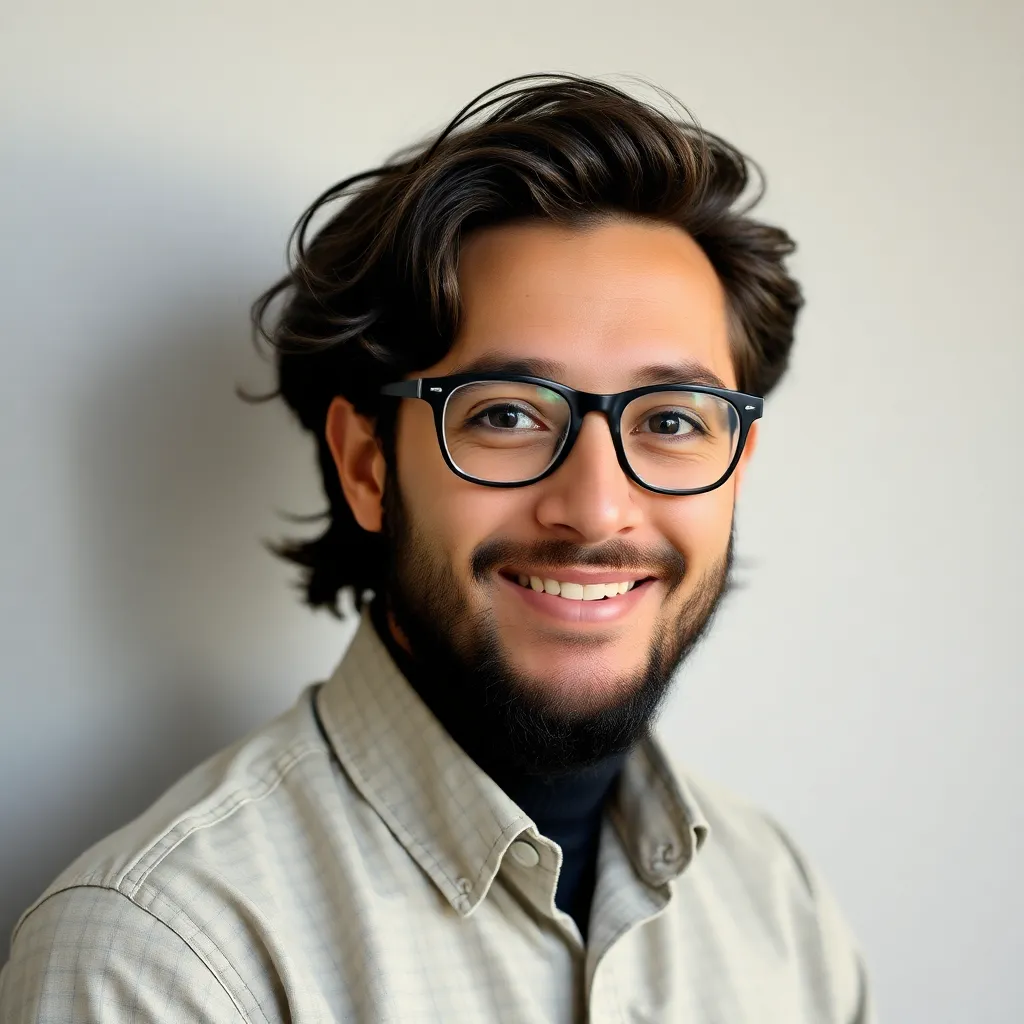
listenit
Apr 25, 2025 · 7 min read

Table of Contents
How Many Valence Electrons Do Transition Metals Have? A Deep Dive
Transition metals, the fascinating elements residing in the d-block of the periodic table, are renowned for their variable oxidation states and vibrant colored compounds. Understanding their electronic structure, specifically the number of valence electrons, is key to unraveling their unique properties and behaviors. This article delves into the complexities of valence electron count in transition metals, exploring the nuances that make them so intriguing.
Defining Valence Electrons and Transition Metals
Before we dive into the specifics, let's establish a clear understanding of our core terms. Valence electrons are the electrons in the outermost shell of an atom that participate in chemical bonding. These electrons are the most loosely held and therefore most readily available for interaction with other atoms. This explains their crucial role in determining an element's chemical reactivity and bonding properties.
Transition metals, also known as transition elements, are the elements found in groups 3 to 12 of the periodic table. They are characterized by the progressive filling of their d orbitals. This incompletely filled d subshell is the primary reason for their unique characteristics, such as variable oxidation states, catalytic activity, and the formation of colorful complexes.
The Complexity of Valence Electron Count in Transition Metals
Unlike main group elements where the number of valence electrons is straightforwardly determined by their group number (e.g., Group 1 elements have one valence electron), transition metals present a more complex scenario. While the ns electrons (where 'n' is the principal quantum number) are generally considered valence electrons, the involvement of the (n-1)d electrons in bonding adds a layer of complexity.
The number of valence electrons isn't always a fixed number for a given transition metal. This is because the energy difference between the ns and (n-1)d orbitals is relatively small. This small energy difference allows for the participation of both ns and (n-1)d electrons in chemical bonding, leading to variable oxidation states.
The Role of the d-Orbitals
The (n-1)d orbitals play a pivotal role in determining the variable oxidation states observed in transition metals. These orbitals can participate in bonding, leading to different numbers of electrons being involved in the chemical bonds formed. This contrasts sharply with main group elements, where the valence electrons primarily reside in the s and p orbitals.
For instance, consider iron (Fe). Its electronic configuration is [Ar] 3d⁶ 4s². While the 4s² electrons are often considered the first to be involved in bonding, the 3d⁶ electrons can also participate, leading to iron exhibiting oxidation states ranging from +2 to +6. This flexibility stems from the relatively small energy difference between the 3d and 4s orbitals.
Determining the Number of Valence Electrons: A Case-by-Case Approach
Due to this complexity, there isn't a single, universally applicable rule for determining the number of valence electrons in transition metals. Instead, a case-by-case approach considering the specific element and its oxidation state is necessary. However, some general guidelines can help:
-
The ns electrons are always considered valence electrons: The electrons in the outermost s orbital are consistently involved in bonding and are considered valence electrons. For most transition metals, this means two valence electrons are initially available.
-
The (n-1)d electrons can participate: The number of (n-1)d electrons that participate in bonding depends on the specific compound and the oxidation state of the transition metal. Some compounds may involve only the ns electrons, while others may utilize both ns and (n-1)d electrons.
-
Oxidation state dictates the number of valence electrons involved in bonding: The oxidation state of a transition metal directly reflects the number of valence electrons involved in chemical bonding. A higher oxidation state suggests a greater number of valence electrons are participating in the bond formation.
Let's look at some specific examples to illustrate this:
-
Iron (Fe): As mentioned earlier, Fe has an electronic configuration of [Ar] 3d⁶ 4s². In its +2 oxidation state (Fe²⁺), it loses the two 4s electrons, effectively having zero valence electrons in this particular state. In its +3 oxidation state (Fe³⁺), it loses the two 4s electrons and one 3d electron, again only giving us the number of valence electrons involved in bonding rather than the total number of valence electrons.
-
Copper (Cu): Copper has an electronic configuration of [Ar] 3d¹⁰ 4s¹. Interestingly, it often exhibits a +1 oxidation state (Cu⁺), losing its 4s electron. In this state, the 3d¹⁰ electrons are not significantly involved in bonding. However, in its +2 oxidation state (Cu²⁺), it may lose both the 4s electron and one 3d electron.
-
Chromium (Cr): Chromium’s configuration is [Ar] 3d⁵ 4s¹. This is an exception to the typical filling pattern. It can exhibit oxidation states ranging from +2 to +6, showcasing the involvement of both 4s and 3d electrons.
Consequences of Variable Valence Electrons
The variable number of valence electrons in transition metals has significant consequences:
-
Variable Oxidation States: This is perhaps the most defining characteristic of transition metals. The ability to lose different numbers of electrons leads to a wide range of oxidation states, resulting in diverse chemical properties and compound formation.
-
Formation of Colored Compounds: The partially filled d orbitals in transition metals allow for the absorption and emission of light in the visible region, resulting in the formation of colorful complexes. This phenomenon is responsible for the striking colors observed in many transition metal compounds.
-
Catalytic Activity: The ability of transition metals to easily change their oxidation states allows them to act as catalysts in numerous chemical reactions. They can accept and donate electrons readily, facilitating the reaction process.
Beyond Simple Counting: A Deeper Look at Bonding
The discussion so far has focused on the number of valence electrons, but it's important to acknowledge the complexities of chemical bonding in transition metals. While the number of valence electrons provides a valuable starting point, the actual bonding behavior is influenced by many factors including:
-
Ligand Field Theory: This theory describes how the d-orbitals of a transition metal ion are affected by the surrounding ligands (atoms, ions, or molecules bound to the metal). The interaction between the metal and ligands significantly influences the energy levels of the d-orbitals, impacting bonding and properties.
-
Crystal Field Theory: A simpler model related to Ligand Field Theory, providing a qualitative understanding of d-orbital splitting and its effect on the properties of transition metal complexes.
-
Molecular Orbital Theory: Provides a more comprehensive description of bonding by considering the combination of atomic orbitals to form molecular orbitals. This approach can be particularly useful for understanding complex transition metal compounds.
Applications and Importance
Understanding the valence electrons of transition metals is crucial in various fields:
-
Catalysis: Numerous industrial processes rely on transition metal catalysts, such as those used in petroleum refining, ammonia synthesis (Haber-Bosch process), and the production of plastics.
-
Materials Science: The unique properties of transition metals make them vital in developing advanced materials, including alloys with specific strength, corrosion resistance, or magnetic properties.
-
Biochemistry: Transition metals play essential roles in various biological systems, often serving as cofactors in enzymes and proteins involved in crucial metabolic processes. Examples include iron in hemoglobin, copper in cytochrome c oxidase, and zinc in many enzymes.
-
Pigments and Dyes: The vibrant colors of transition metal compounds make them highly valued in the production of pigments and dyes for paints, textiles, and cosmetics.
Conclusion: The Enigmatic Nature of Transition Metal Valence Electrons
Determining the exact number of valence electrons in transition metals is not a straightforward task. The small energy difference between the ns and (n-1)d orbitals allows for variable participation of these electrons in bonding, leading to the characteristic variable oxidation states and diverse chemical properties of these fascinating elements. A deeper understanding necessitates the application of more sophisticated bonding theories like Ligand Field Theory and Molecular Orbital Theory. Despite the complexity, the fundamental concept of valence electrons remains a cornerstone in understanding the reactivity, bonding behavior, and numerous applications of transition metals in various scientific and technological fields. Continued research continues to refine our understanding of these intricate elements and their crucial roles in the world around us.
Latest Posts
Latest Posts
-
Atoms Of The Same Element Can Have Different Properties
Apr 28, 2025
-
5 Percent As A Fraction In Simplest Form
Apr 28, 2025
-
What Is The Basic Metric Unit Of Weight
Apr 28, 2025
-
Which Transition Causes The Absorption Line At The Shortest Wavelength
Apr 28, 2025
-
Atomic Number Is Equal To The Number Of
Apr 28, 2025
Related Post
Thank you for visiting our website which covers about How Many Valence Electrons Does Transition Metals Have . We hope the information provided has been useful to you. Feel free to contact us if you have any questions or need further assistance. See you next time and don't miss to bookmark.