How Many Electrons Can The D Orbital Hold
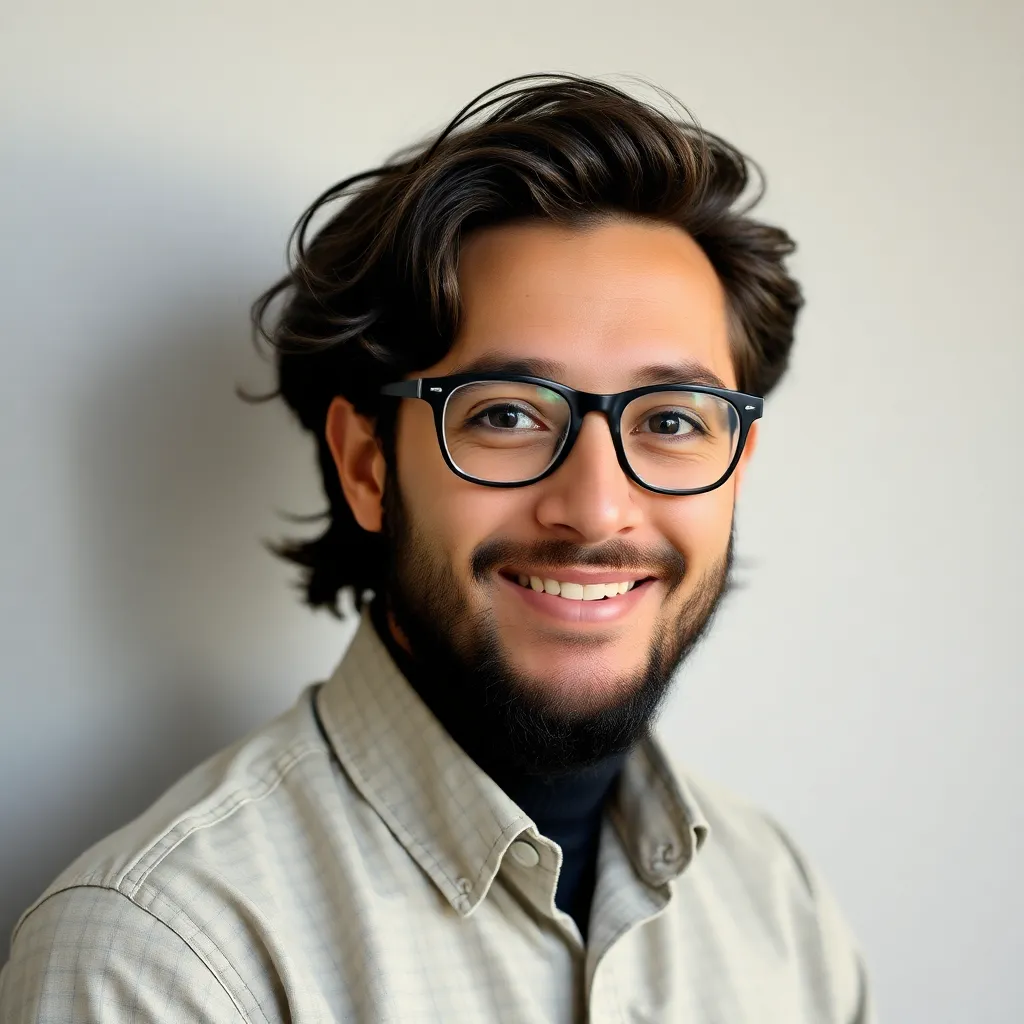
listenit
Mar 10, 2025 · 6 min read

Table of Contents
How Many Electrons Can the d Orbital Hold? A Deep Dive into Atomic Structure
Understanding the electron configuration of atoms is fundamental to chemistry. This article delves into the specifics of the d orbital, explaining its capacity for electrons and the principles governing its behavior. We'll explore the quantum numbers that define the d orbital, its shape, and the role it plays in chemical bonding and the periodic table. By the end, you’ll have a comprehensive understanding of why the d orbital holds the number of electrons it does.
Understanding Electron Orbitals: A Quick Recap
Before we dive into the specifics of the d orbital, let's briefly review the basics of electron orbitals. Electrons don't orbit the nucleus in neat, predictable paths like planets around a star. Instead, they exist in regions of space called orbitals, which are defined by their probability density – the likelihood of finding an electron within a particular volume.
These orbitals are described by four quantum numbers:
-
Principal quantum number (n): This determines the energy level of the electron and the size of the orbital. It can be any positive integer (1, 2, 3, etc.).
-
Azimuthal quantum number (l): This defines the shape of the orbital and its angular momentum. It can range from 0 to n - 1. Specific values of l correspond to different orbital types:
- l = 0: s orbital (spherical)
- l = 1: p orbital (dumbbell-shaped)
- l = 2: d orbital (more complex shapes)
- l = 3: f orbital (even more complex shapes)
-
Magnetic quantum number (ml): This specifies the orientation of the orbital in space. It can range from -l to +l, including 0. For example, a p orbital (l = 1) has three possible orientations (ml = -1, 0, +1).
-
Spin quantum number (ms): This describes the intrinsic angular momentum of the electron, often referred to as "spin." It can have only two values: +1/2 (spin up) or -1/2 (spin down). This is crucial for understanding how electrons fill orbitals.
The d Orbital: Shape and Degeneracy
The d orbital is defined by the azimuthal quantum number l = 2. This means it has five possible orientations in space, corresponding to five different d orbitals. These orbitals are often designated as d<sub>xy</sub>, d<sub>xz</sub>, d<sub>yz</sub>, d<sub>x²−y²</sub>, and d<sub>z²</sub>. Their shapes are more complex than s or p orbitals, featuring multiple lobes and nodal planes (regions where the probability of finding an electron is zero).
The five d orbitals within a given principal energy level (n) are degenerate, meaning they have the same energy. However, this degeneracy can be lifted (the orbitals can have slightly different energies) in the presence of a ligand field, as seen in coordination chemistry. This splitting of d orbital energies is crucial for understanding the properties of transition metal complexes.
How Many Electrons Can a Single d Orbital Hold?
According to the Pauli Exclusion Principle, no two electrons in an atom can have the same set of four quantum numbers. Since each d orbital has a specific set of (n, l, ml), it can accommodate a maximum of two electrons, one with spin up (+1/2) and one with spin down (-1/2).
This principle is paramount. It's not just about spatial arrangement within the orbital's shape, but also the fundamental quantum nature of electrons themselves. They can't simply pile up, regardless of available space; their quantum states must be distinct.
How Many Electrons Can the Entire d Subshell Hold?
Because there are five d orbitals in a d subshell, and each orbital can hold two electrons, the entire d subshell can hold a maximum of ten electrons (5 orbitals x 2 electrons/orbital = 10 electrons). This is a crucial number in understanding the electron configurations of transition metals, which are characterized by partially filled d orbitals.
This ten-electron capacity is reflected in the periodic table's structure. The transition metal series are located where the d orbitals are progressively filled. The properties of these elements, such as their variable oxidation states and colorful complexes, are directly related to the presence of electrons in their d orbitals.
Filling d Orbitals: Hund's Rule and Electron Configuration
When filling d orbitals (or any orbitals), we follow Hund's Rule. Hund's Rule states that electrons will individually occupy each orbital within a subshell before doubling up in any one orbital. This minimizes electron-electron repulsion and results in a more stable electron configuration.
For example, consider chromium (Cr), which has an atomic number of 24. One might expect its electron configuration to be [Ar] 3d<sup>4</sup> 4s<sup>2</sup>. However, due to Hund's Rule and the relatively small energy difference between the 3d and 4s orbitals, one electron from the 4s orbital actually jumps to the 3d orbital to maximize the number of unpaired electrons. This leads to a more stable configuration: [Ar] 3d<sup>5</sup> 4s<sup>1</sup>. This exemplifies the nuanced and sometimes counterintuitive behavior of electrons in filling orbitals.
The Importance of d Orbitals in Chemistry
The d orbitals play a vital role in a broad range of chemical phenomena, including:
-
Transition metal chemistry: Transition metals exhibit diverse oxidation states and form complex ions due to the involvement of d electrons in bonding. This leads to catalytic activity, vibrant colors, and magnetic properties. Examples abound, from enzymes containing transition metals to the use of transition metal catalysts in industrial processes.
-
Coordination complexes: The bonding in coordination complexes is explained by the interaction of ligands (molecules or ions) with the d orbitals of the central metal ion. The crystal field theory and ligand field theory, which detail this interaction, are essential concepts in inorganic chemistry. The colors of these complexes, for example, often arise from d-d electron transitions.
-
Catalysis: Many important catalytic reactions rely on transition metals, and the d orbitals are central to their catalytic activity. These metals can facilitate reactions by accepting or donating electrons, acting as electron sinks or reservoirs. Examples include catalytic converters in automobiles and enzymes that use transition metals as active sites.
-
Spectroscopy: The electronic transitions involving d orbitals are responsible for the characteristic colors of many transition metal compounds. This is exploited in spectroscopic techniques like UV-Vis spectroscopy, which is widely used to identify and quantify these compounds. The absorption of light promotes electrons within the d subshell to higher energy levels.
Conclusion: The Significance of the d Orbital Electron Capacity
The fact that a d orbital can hold a maximum of two electrons, and a d subshell can hold ten, is not a mere mathematical curiosity. It's a fundamental principle that underpins the behavior of countless chemical systems. Understanding this capacity and the related principles like the Pauli Exclusion Principle and Hund's Rule provides a crucial foundation for grasping the properties of transition metals, the formation of coordination complexes, and various catalytic processes. The d orbitals' influence reaches far beyond simple atomic structure, shaping the vibrant colors of gemstones, the effectiveness of industrial catalysts, and even the mechanisms of life itself. This knowledge highlights the depth and interconnectedness of chemical principles and their profound impact on the world around us.
Latest Posts
Latest Posts
-
Why Is The Plasma Membrane Called A Selectively Permeable
May 09, 2025
-
Is A Boiling Point A Physical Property
May 09, 2025
-
3 Gallon Bucket And 5 Gallon Bucket Riddle
May 09, 2025
-
Primary Spermatocyte Is Haploid Or Diploid
May 09, 2025
-
When Dissolved In Water Bases Produce
May 09, 2025
Related Post
Thank you for visiting our website which covers about How Many Electrons Can The D Orbital Hold . We hope the information provided has been useful to you. Feel free to contact us if you have any questions or need further assistance. See you next time and don't miss to bookmark.