How Is The Modern Atomic Model Different From Bohr's Model
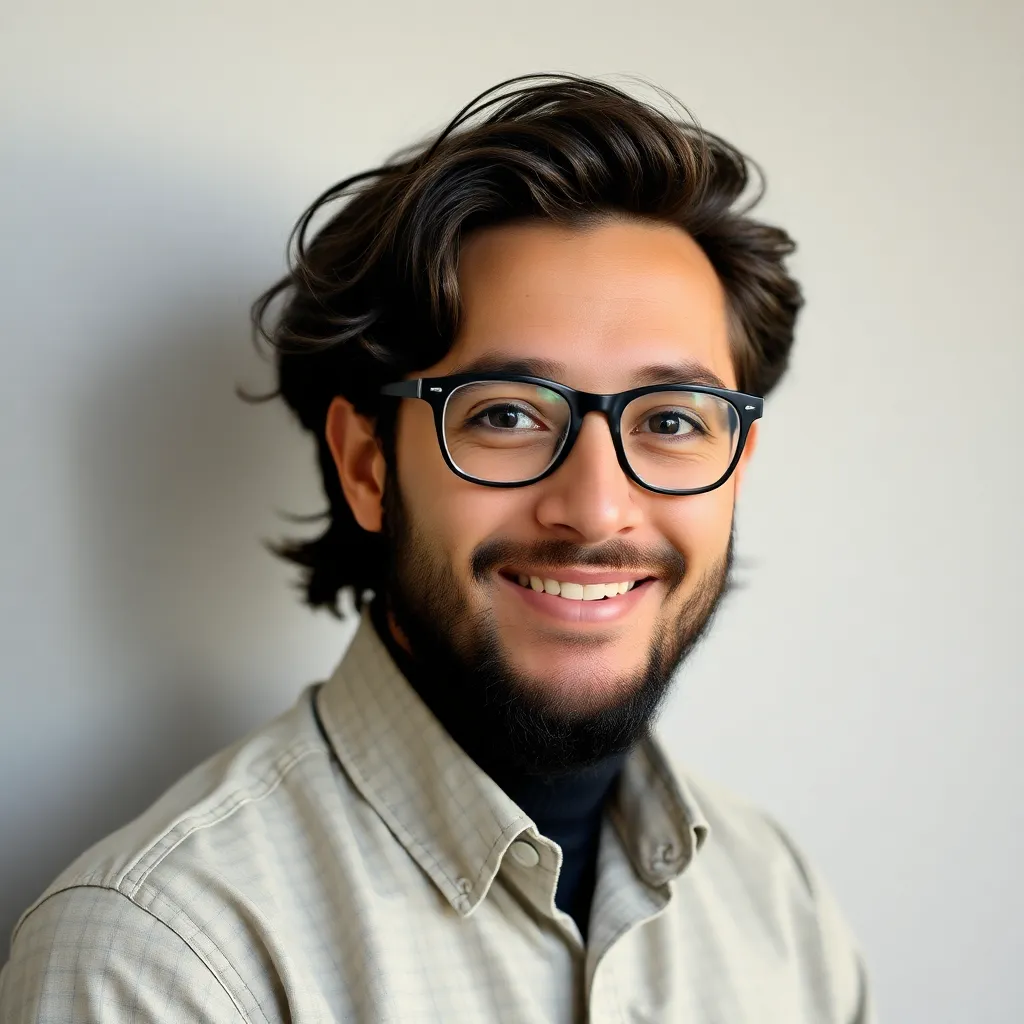
listenit
Apr 26, 2025 · 7 min read

Table of Contents
How is the Modern Atomic Model Different from Bohr's Model?
The atom, the fundamental building block of matter, has been the subject of intense scientific scrutiny for centuries. Our understanding has evolved dramatically, from the initial concept of indivisible particles to the complex quantum mechanical models we use today. A pivotal step in this journey was Niels Bohr's model in 1913, which revolutionized atomic theory. However, Bohr's model, while a significant advancement, possessed limitations that necessitated further refinement, leading to the development of the modern atomic model. This article will delve into the key differences between these two models, highlighting the advancements and complexities introduced by quantum mechanics.
Bohr's Model: A Revolutionary Leap
Bohr's model, proposed in 1913, offered a significant improvement over previous models. It successfully explained the observed line spectra of hydrogen, a phenomenon that earlier models failed to account for. Bohr's model incorporated two revolutionary postulates:
Postulate 1: Quantized Orbits
Bohr postulated that electrons revolve around the nucleus in specific, quantized orbits. This meant electrons could only exist in certain discrete energy levels, rather than at any arbitrary distance from the nucleus. Each orbit corresponds to a specific energy level, with lower orbits representing lower energy states. This was a radical departure from classical physics, which predicted that electrons orbiting a nucleus would continuously emit radiation, spiraling into the nucleus and causing the atom to collapse.
Postulate 2: Quantum Jumps
Bohr proposed that electrons can only transition between these quantized orbits by absorbing or emitting photons of specific energies. The energy of the photon precisely matches the energy difference between the initial and final orbits. This explained the discrete lines in the hydrogen spectrum, with each line corresponding to a specific electron transition between energy levels. This concept of "quantum jumps" laid the foundation for the understanding of atomic transitions and spectral lines.
Limitations of Bohr's Model
Despite its groundbreaking success with the hydrogen atom, Bohr's model suffered from several limitations:
Inaccurate for Multi-electron Atoms
Bohr's model was primarily successful in explaining the hydrogen atom's spectrum, which possesses only one electron. Applying the model to multi-electron atoms proved significantly more challenging. The interactions between multiple electrons couldn't be accurately described using Bohr's simple model, leading to discrepancies between predicted and observed spectral lines. The model failed to account for the electron-electron repulsion accurately.
Failed to Explain Fine Structure
The spectral lines of even hydrogen, when examined closely, exhibit a fine structure—a subtle splitting of lines into multiple closely spaced components. Bohr's model couldn't explain this phenomenon, highlighting the limitations of its simplistic approach. The fine structure is due to relativistic effects and spin-orbit coupling, aspects not incorporated into Bohr's model.
No Explanation for Chemical Bonding
Bohr's model offered little insight into chemical bonding, a crucial aspect of chemical behavior. While the model provided a framework for understanding electron arrangements within atoms, it lacked the tools to explain how atoms interact and form molecules.
Could Not Explain Zeeman Effect and Stark Effect
The Zeeman effect (splitting of spectral lines in a magnetic field) and the Stark effect (splitting of spectral lines in an electric field) are further phenomena that Bohr's model could not satisfactorily explain. These effects arise from the interaction of the electron's orbital angular momentum with external fields, complexities not incorporated into Bohr's model.
The Modern Atomic Model: Quantum Mechanics Takes Center Stage
The inadequacies of Bohr's model paved the way for the development of the modern atomic model, based on the principles of quantum mechanics. Quantum mechanics offers a far more sophisticated and accurate description of atomic behavior. Here are the key differences:
Wave-Particle Duality
The modern atomic model embraces the wave-particle duality of electrons, a concept absent from Bohr's model. Electrons behave both as particles and as waves. This dual nature is described by the electron's wave function, a mathematical function that describes the probability of finding the electron at a particular location in space. The electron's location is probabilistic, not deterministic as suggested by Bohr's model.
Uncertainty Principle
Heisenberg's uncertainty principle is a cornerstone of the modern atomic model. It states that it is impossible to simultaneously know both the position and momentum of an electron with perfect accuracy. This inherent uncertainty has profound implications for our understanding of electron behavior within atoms. It highlights the limitations of the classical picture of electrons orbiting the nucleus in precisely defined paths.
Orbitals Replace Orbits
The modern atomic model replaces Bohr's concept of well-defined orbits with orbitals. An orbital is a region of space where there is a high probability of finding an electron. Unlike Bohr's orbits, orbitals are not well-defined paths but rather probability distributions. This reflects the inherent uncertainty in the electron's location. Orbitals are characterized by quantum numbers (n, l, ml, ms), which specify the energy level, shape, orientation, and spin of the electron.
Quantum Numbers
The modern model incorporates a more detailed and complete set of quantum numbers compared to Bohr's simplified approach. These quantum numbers provide a more comprehensive description of the electron's state:
- Principal Quantum Number (n): Determines the energy level and size of the orbital.
- Azimuthal Quantum Number (l): Determines the shape of the orbital (s, p, d, f, etc.).
- Magnetic Quantum Number (ml): Determines the orientation of the orbital in space.
- Spin Quantum Number (ms): Describes the intrinsic angular momentum (spin) of the electron.
These quantum numbers provide a far richer description of electron behavior compared to Bohr's simplified model.
Electron Configuration and the Aufbau Principle
The modern model explains the arrangement of electrons within an atom using electron configuration and the Aufbau principle. The Aufbau principle states that electrons fill orbitals in order of increasing energy. This explains the periodic trends observed in the periodic table. The electron configuration provides information on the number of electrons in each subshell.
Accurate Predictions for Multi-electron Atoms
The modern model, based on quantum mechanics, provides far more accurate predictions for the spectral lines of multi-electron atoms than Bohr's model. It accounts for electron-electron interactions more effectively, leading to a better agreement between theory and experiment.
Explanation of Chemical Bonding
The modern atomic model provides a robust framework for understanding chemical bonding. The concept of orbitals and electron configurations allows us to explain the formation of covalent and ionic bonds. Molecular orbital theory, a direct consequence of the modern atomic model, successfully predicts the properties of molecules.
Beyond the Basics: More Complex Phenomena
The modern atomic model extends far beyond explaining the basic structure of atoms. It incorporates more nuanced and complex phenomena:
Relativistic Effects
For heavier atoms, relativistic effects become increasingly significant. These effects arise from the high speeds of inner-shell electrons and require relativistic corrections to the Schrödinger equation. The modern model accounts for these relativistic effects, which influence the chemical properties of heavy elements.
Quantum Electrodynamics (QED)
QED is a more complete theory that combines quantum mechanics and electromagnetism. It explains the interaction of electrons and photons with high precision. QED is essential for an even more accurate understanding of atomic structure and spectra.
Conclusion: A Paradigm Shift in Atomic Theory
The modern atomic model represents a paradigm shift from Bohr's model. While Bohr's model provided a crucial stepping stone in our understanding of the atom, its limitations necessitated the development of a more sophisticated model based on quantum mechanics. The modern atomic model, encompassing wave-particle duality, the uncertainty principle, orbitals, and quantum numbers, provides a far more accurate and comprehensive picture of atomic structure and behavior. It successfully explains a wider range of phenomena, from the fine structure of spectral lines to the complexities of chemical bonding in multi-electron atoms, establishing a robust framework for understanding the fundamental building blocks of matter. The ongoing advancements in quantum mechanics continue to refine our understanding, pushing the boundaries of what we know about the atom and its behavior.
Latest Posts
Latest Posts
-
What Is 44 Percent Of 50
Apr 27, 2025
-
Does Nacl Have A High Melting Point
Apr 27, 2025
-
3 And 2 5 As An Improper Fraction
Apr 27, 2025
-
Can Mitochondria Be Seen With A Light Microscope
Apr 27, 2025
-
What Is 8 Divided By 64
Apr 27, 2025
Related Post
Thank you for visiting our website which covers about How Is The Modern Atomic Model Different From Bohr's Model . We hope the information provided has been useful to you. Feel free to contact us if you have any questions or need further assistance. See you next time and don't miss to bookmark.