How Does Atomic Radius Increase Across The Periodic Table
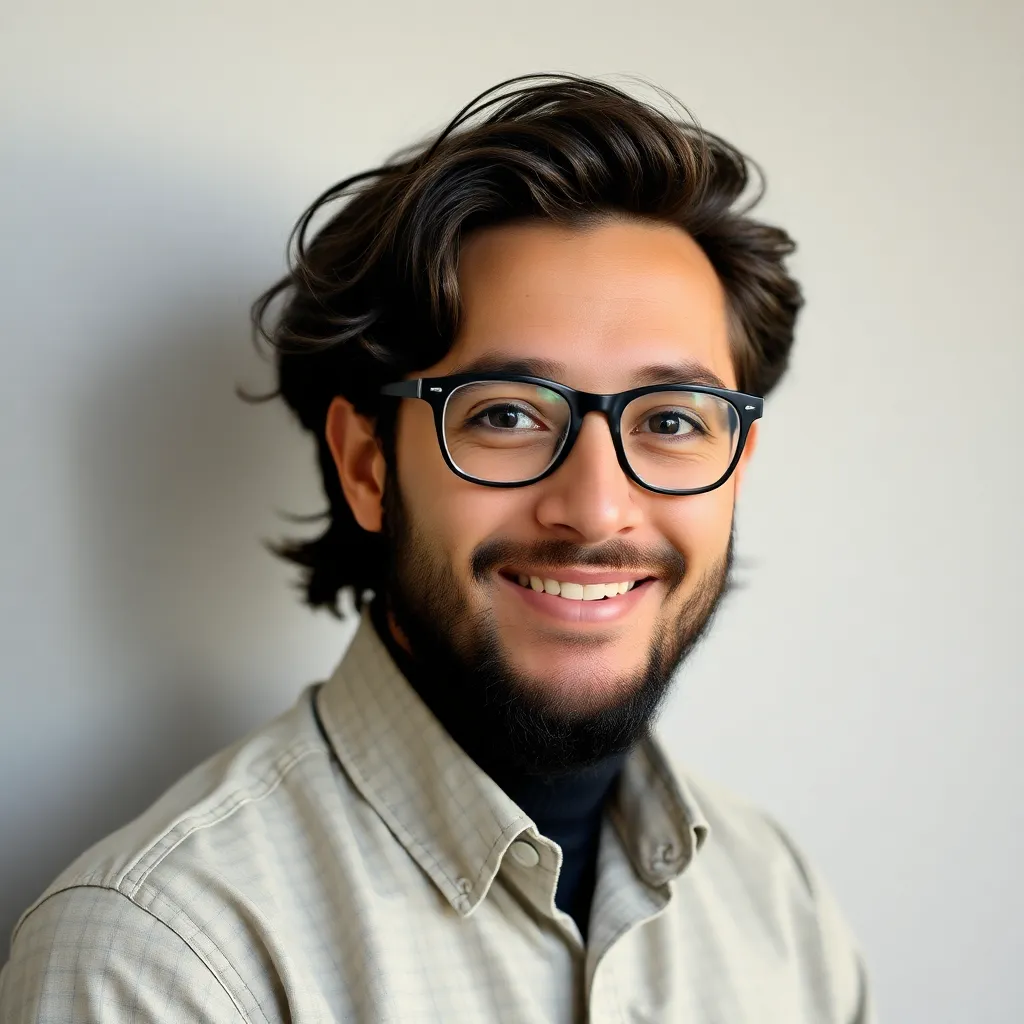
listenit
Apr 05, 2025 · 6 min read
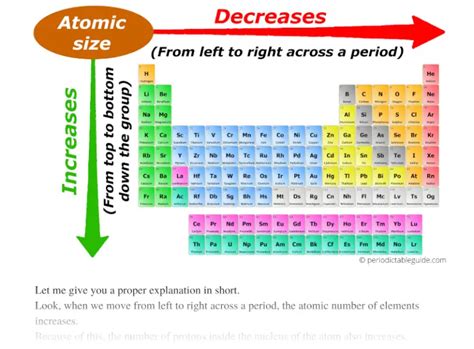
Table of Contents
How Does Atomic Radius Increase Across the Periodic Table?
Understanding atomic radius trends across the periodic table is fundamental to grasping the behavior of elements and their interactions. This seemingly simple concept underpins a wide range of chemical and physical properties, from reactivity to conductivity. This comprehensive guide will delve deep into the factors influencing atomic radius, explaining the trends observed across periods and down groups, and addressing common misconceptions.
What is Atomic Radius?
Before exploring trends, we need a clear definition. Atomic radius refers to the distance from the atom's nucleus to its outermost stable electron. It's crucial to remember that this isn't a fixed, easily measurable distance. Electrons exist in orbitals, regions of probability rather than precise locations. Therefore, atomic radius is often defined as half the distance between the nuclei of two identical atoms bonded together. This approach provides a more practical and consistent measure.
Factors Affecting Atomic Radius
Several key factors influence the size of an atom:
1. Number of Protons (Nuclear Charge):
The positive charge of the nucleus, determined by the number of protons, strongly attracts the negatively charged electrons. A higher nuclear charge pulls electrons closer to the nucleus, resulting in a smaller atomic radius. This effect is prominent across a period (row) of the periodic table, where the number of protons increases while the energy level of the outermost electrons remains the same.
2. Number of Electron Shells (Energy Levels):
As we move down a group (column) in the periodic table, the number of electron shells increases. Each shell represents a higher energy level, and electrons in these outer shells are further from the nucleus. Therefore, adding electron shells significantly increases the atomic radius. This effect outweighs the increased nuclear charge within a group, leading to a larger atomic radius as we go down.
3. Shielding Effect:
Inner electrons shield outer electrons from the full positive charge of the nucleus. This shielding effect reduces the effective nuclear charge experienced by the outermost electrons. The more inner electrons present, the greater the shielding effect, leading to a weaker attraction between the nucleus and the outer electrons, and thus a larger atomic radius. This is a significant factor when comparing atoms within a group.
4. Electron-Electron Repulsion:
Electrons within the same shell repel each other. This electron-electron repulsion counteracts the attractive force from the nucleus. As the number of electrons in a shell increases, the repulsion increases, causing the outer electrons to spread out, slightly increasing the atomic radius. This effect is relatively smaller compared to the influence of nuclear charge and shielding.
Trends in Atomic Radius Across the Periodic Table
Now let's examine how these factors play out in the observed trends:
Across a Period (Left to Right):
Moving from left to right across a period, the atomic radius generally decreases. This is primarily due to the increasing nuclear charge. While additional electrons are added, they are all placed in the same energy level (shell). The increased positive charge of the nucleus effectively pulls the electrons closer, overriding the slight increase in electron-electron repulsion. The shielding effect remains relatively constant as the number of inner electrons doesn't change significantly within a period.
Example: Consider the period starting with Lithium (Li) and ending with Neon (Ne). Lithium has a smaller nuclear charge and fewer electrons, leading to a larger atomic radius compared to Neon.
Down a Group (Top to Bottom):
Moving down a group, the atomic radius generally increases. The dominant factor here is the addition of electron shells. Each new shell significantly increases the distance between the nucleus and the outermost electrons. Although the nuclear charge also increases, the shielding effect from the inner electrons mitigates this increase in positive charge, preventing a significant decrease in the atomic radius.
Example: Consider the alkali metals (Group 1). As we move from Lithium (Li) to Francium (Fr), the atomic radius increases considerably due to the addition of multiple electron shells.
Exceptions and Anomalies
While the general trends are clear, there are some exceptions and anomalies that need consideration:
-
Transition Metals: The atomic radii of transition metals show less significant variation across a period compared to the main group elements. This is because electrons are added to inner d-orbitals, which are less effective at shielding the outermost electrons than s and p orbitals. The increased nuclear charge is partially compensated by the inner d-electrons, resulting in relatively smaller changes in atomic size.
-
Lanthanides and Actinides: These elements exhibit a unique phenomenon called the lanthanide contraction and actinide contraction, respectively. The poor shielding effect of the f-orbitals leads to a greater-than-expected increase in effective nuclear charge, causing a smaller-than-expected increase in atomic radius across these series.
-
Anomalous Pairs: Certain pairs of elements show slight deviations from the expected trend. These deviations often involve subtle differences in electron configuration or inter-electronic repulsion.
Applications and Significance of Atomic Radius Trends
Understanding atomic radius trends has significant implications across various fields:
-
Chemical Reactivity: Atomic size directly influences an atom's ability to gain or lose electrons, significantly affecting its reactivity. Smaller atoms generally have a higher ionization energy and electron affinity, making them less likely to lose or gain electrons.
-
Bonding: Atomic radius plays a crucial role in determining the type of bond formed between atoms (ionic, covalent, metallic). Larger atoms with low ionization energies tend to form ionic bonds, while smaller atoms with high ionization energies form covalent bonds.
-
Physical Properties: Atomic radius impacts properties like melting point, boiling point, density, and electrical conductivity. These properties are intricately linked to the arrangement and interactions of atoms.
-
Material Science: Atomic radius knowledge is indispensable in designing new materials with specific properties. For example, understanding atomic size enables researchers to tailor materials for specific applications by manipulating their structure and composition.
Conclusion
The periodic trend of atomic radius is a cornerstone of chemistry. Understanding the interplay of nuclear charge, shielding, and electron shells allows us to predict and explain variations in atomic size across the periodic table. These trends have far-reaching consequences, affecting the chemical and physical properties of elements and their compounds, and ultimately shaping our understanding of the material world. Although some exceptions and anomalies exist, the general trends provide a powerful framework for predicting atomic behavior and informing research in diverse fields. Continued exploration of atomic radius and its related concepts remains crucial for advancements in chemistry, materials science, and related disciplines. This fundamental principle continues to be a source of fascinating research and discovery.
Latest Posts
Latest Posts
-
Which Element Has Chemical Properties Most Similar To Sodium
Apr 05, 2025
-
What Shape Has 4 Right Angles
Apr 05, 2025
-
Dna Replication Is Said To Be Semiconservative Because
Apr 05, 2025
-
Why Is A Graduated Cylinder More Accurate Than A Beaker
Apr 05, 2025
-
What Is The Molecular Mass Of Iron
Apr 05, 2025
Related Post
Thank you for visiting our website which covers about How Does Atomic Radius Increase Across The Periodic Table . We hope the information provided has been useful to you. Feel free to contact us if you have any questions or need further assistance. See you next time and don't miss to bookmark.