How Does An Enzyme Affect A Chemical Reaction
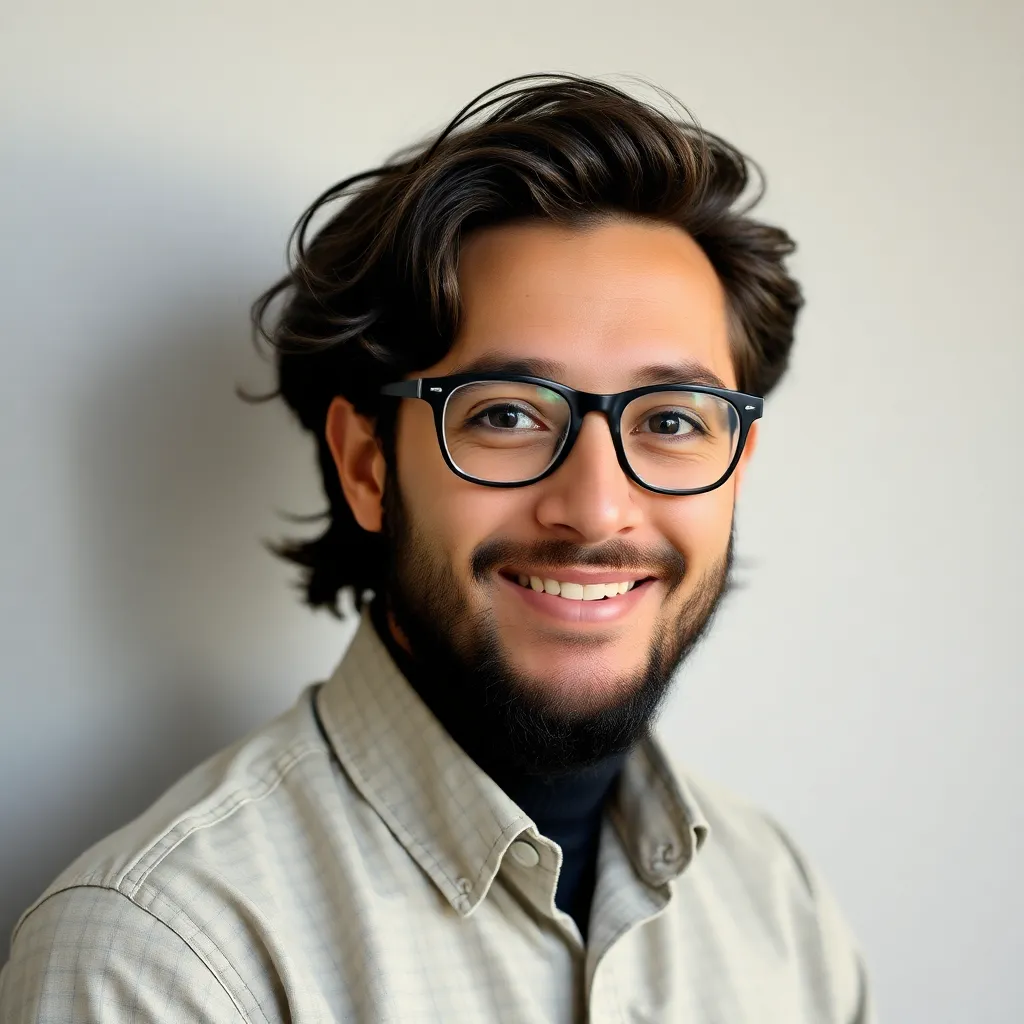
listenit
Mar 28, 2025 · 7 min read
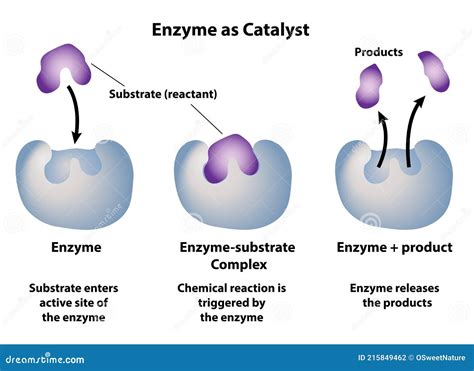
Table of Contents
How Do Enzymes Affect Chemical Reactions?
Enzymes are biological catalysts, which means they speed up chemical reactions without being consumed in the process. Understanding how enzymes achieve this remarkable feat is crucial to comprehending the fundamental workings of life itself. From digestion to DNA replication, enzymes orchestrate the complex chemical processes that sustain all living organisms. This article delves deep into the mechanisms by which enzymes influence chemical reactions, exploring their structure, function, and the factors that affect their activity.
The Nature of Enzymes: Biological Catalysts
Before diving into the mechanics of enzyme action, it's essential to understand what makes enzymes unique. Unlike inorganic catalysts, enzymes are proteins, folded into intricate three-dimensional structures. This precise arrangement is critical to their function, as it creates an active site – a specific region where the enzyme interacts with its substrate (the molecule the enzyme acts upon). The active site possesses a unique shape and chemical environment perfectly tailored to bind with the substrate and facilitate the reaction.
Key characteristics of enzymes as biological catalysts:
- Specificity: Enzymes are highly specific, meaning they typically catalyze only one particular reaction or a very limited set of related reactions. This specificity arises from the precise fit between the enzyme's active site and its substrate. This "lock and key" model, while a simplification, highlights the crucial role of enzyme shape.
- Efficiency: Enzymes significantly increase the rate of chemical reactions, often by many orders of magnitude. This remarkable efficiency is due to their ability to lower the activation energy of the reaction.
- Regulation: Enzyme activity is often tightly regulated, allowing cells to control metabolic pathways and respond to changing conditions. This regulation can involve various mechanisms, such as allosteric regulation, feedback inhibition, and covalent modification.
- Biodegradability: Enzymes are biodegradable, meaning they are eventually broken down by other enzymes within the cell or organism. This contrasts with many inorganic catalysts, which are often persistent pollutants.
The Mechanism of Enzyme Action: Lowering Activation Energy
The core function of an enzyme is to lower the activation energy (Ea) of a chemical reaction. Activation energy represents the minimum energy required for a reaction to proceed. By lowering this energy barrier, enzymes allow reactions to occur much faster at a given temperature. This is achieved primarily through several mechanisms:
1. Substrate Binding and Orientation: The Induced Fit Model
The initial interaction between an enzyme and its substrate often involves a process described by the "induced fit" model. This model refines the older "lock and key" model, recognizing that the enzyme's active site is not a rigid structure but rather a flexible one. Upon substrate binding, the active site undergoes a conformational change, optimizing the interaction and positioning the substrate ideally for the reaction. This precise orientation of the substrate molecules significantly increases the likelihood of successful collisions leading to product formation.
2. Stabilizing the Transition State: Proximity and Orientation Effects
Once the substrate is bound to the active site, the enzyme actively participates in the reaction by stabilizing the transition state. The transition state is a high-energy, unstable intermediate formed during the conversion of substrate to product. Enzymes stabilize this transition state by a variety of means:
- Proximity and Orientation: By bringing the substrate molecules into close proximity and aligning them correctly, the enzyme increases the probability of the reaction occurring. This effect is particularly important for bimolecular reactions, where two substrates must collide to react.
- Acid-Base Catalysis: Certain amino acid residues within the active site can act as acids or bases, donating or accepting protons to facilitate bond breaking or formation.
- Covalent Catalysis: The enzyme may form a temporary covalent bond with the substrate, creating a more reactive intermediate that subsequently leads to product formation.
- Metal Ion Catalysis: Some enzymes require metal ions (e.g., zinc, magnesium, iron) for their catalytic activity. These ions can participate in the reaction by stabilizing negative charges, mediating redox reactions, or facilitating substrate binding.
3. Product Release and Enzyme Regeneration
Once the reaction is complete, the enzyme releases the product(s) and returns to its original conformation, ready to catalyze another reaction. This cycle of substrate binding, catalysis, and product release continues as long as there is substrate available and the enzyme remains functional.
Factors Affecting Enzyme Activity
Several factors can influence the rate at which an enzyme catalyzes a reaction. Understanding these factors is crucial for manipulating enzyme activity in various contexts, including industrial processes and medical treatments.
1. Substrate Concentration: The Saturation Curve
At low substrate concentrations, the reaction rate is directly proportional to the substrate concentration. However, as the substrate concentration increases, the rate eventually plateaus. This occurs because the enzyme becomes saturated—all the active sites are occupied by substrate molecules. The maximum rate achieved under saturating conditions is known as Vmax.
2. Temperature: The Optimal Temperature Range
Enzymes have an optimal temperature at which they function most efficiently. At lower temperatures, the reaction rate is slower due to reduced kinetic energy. At higher temperatures, the enzyme can denature – its three-dimensional structure is disrupted, leading to a loss of activity. The optimal temperature varies depending on the specific enzyme and the organism from which it originates.
3. pH: The Optimal pH Range
Similar to temperature, enzymes also have an optimal pH range. Changes in pH can alter the charge distribution on the enzyme's surface and within the active site, affecting its ability to bind substrate and catalyze the reaction. Extreme pH values can denature the enzyme.
4. Inhibitors: Competitive and Non-competitive Inhibition
Enzyme activity can be inhibited by various molecules. Inhibitors can be broadly classified as competitive or non-competitive:
- Competitive Inhibitors: These inhibitors compete with the substrate for binding to the enzyme's active site. The effect of a competitive inhibitor can be overcome by increasing the substrate concentration.
- Non-competitive Inhibitors: These inhibitors bind to a site other than the active site (allosteric site), causing a conformational change in the enzyme that reduces its catalytic activity. The effect of a non-competitive inhibitor cannot be overcome by increasing the substrate concentration.
5. Activators: Enhancing Enzyme Activity
Some enzymes require activators for their activity. Activators can bind to the enzyme and induce a conformational change that enhances its catalytic ability. These activators can be metal ions or other small molecules.
Enzyme Classification and Applications
Enzymes are classified into six broad categories based on the type of reaction they catalyze: oxidoreductases, transferases, hydrolases, lyases, isomerases, and ligases. This classification system provides a framework for understanding the diverse roles enzymes play in biological systems.
The diverse range of enzyme functions translates into a wide array of applications in various fields:
- Medicine: Enzymes are used in diagnostics, therapeutics, and drug development. For example, enzymes are used to diagnose heart attacks and other medical conditions. Enzymes are also being developed as therapeutic agents for various diseases.
- Industry: Enzymes are used in various industrial processes, such as food processing, textile manufacturing, and biofuel production. For instance, enzymes are used to produce high-fructose corn syrup and other sweeteners.
- Agriculture: Enzymes are used in agriculture to enhance nutrient availability in soil and improve crop yields. For example, enzymes are used to break down complex organic matter in soil, releasing nutrients that are accessible to plants.
- Environmental remediation: Enzymes are used in environmental remediation to break down pollutants and clean up contaminated sites.
Conclusion
Enzymes are remarkable biological catalysts that are essential for life. Their ability to significantly accelerate chemical reactions, coupled with their remarkable specificity and regulation, underpins the complexity and efficiency of cellular processes. Understanding how enzymes affect chemical reactions is critical not only for basic biological research but also for developing new technologies in medicine, industry, and environmental science. Further research into enzyme structure, function, and regulation promises to unlock even greater potential for leveraging these extraordinary biomolecules for the benefit of humankind.
Latest Posts
Latest Posts
-
What Is 65 As A Fraction
Mar 31, 2025
-
What Number Is 60 Of 145
Mar 31, 2025
-
The Ability Of An Organism To Survive And Reproduce
Mar 31, 2025
-
What Is A Master Level Clinician
Mar 31, 2025
-
Religion In The Colony Of Virginia
Mar 31, 2025
Related Post
Thank you for visiting our website which covers about How Does An Enzyme Affect A Chemical Reaction . We hope the information provided has been useful to you. Feel free to contact us if you have any questions or need further assistance. See you next time and don't miss to bookmark.