How Do You Balance N2 H2 Nh3
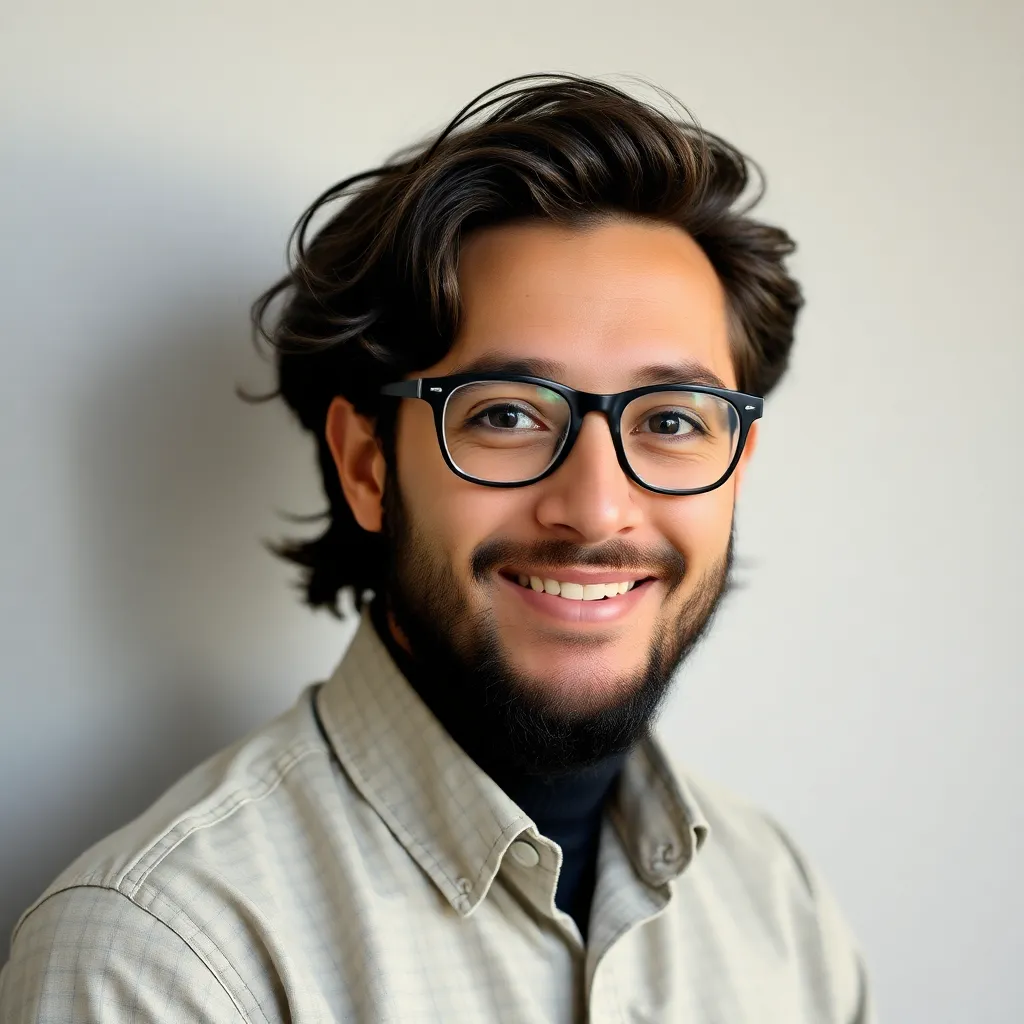
listenit
Apr 24, 2025 · 5 min read

Table of Contents
How Do You Balance N₂ + H₂ → NH₃? A Deep Dive into the Haber-Bosch Process and Reaction Balancing
The synthesis of ammonia (NH₃) from nitrogen (N₂) and hydrogen (H₂) is a cornerstone of modern chemical industry, vital for fertilizer production and numerous other applications. This reaction, famously known as the Haber-Bosch process, is not simply a matter of mixing the gases; it requires careful balancing of the chemical equation and precise control of reaction conditions to achieve optimal yields. This article will delve deep into understanding how to balance the equation N₂ + H₂ → NH₃, exploring the underlying chemistry, the importance of balancing, and the practical implications of this crucial industrial process.
Understanding the Reaction: N₂ + H₂ → NH₃
The reaction between nitrogen and hydrogen to form ammonia is an example of a combination reaction, where two or more substances combine to form a single product. The unbalanced equation, N₂ + H₂ → NH₃, simply represents the reactants and products. However, this representation is incomplete without balancing the equation to reflect the law of conservation of mass. This law states that matter cannot be created or destroyed in a chemical reaction; the total mass of the reactants must equal the total mass of the products.
The Importance of Balancing Chemical Equations
Balancing a chemical equation ensures that the number of atoms of each element is the same on both the reactant and product sides. This is crucial because:
- It reflects the reality of chemical reactions: Chemical reactions involve the rearrangement of atoms, not the creation or destruction of atoms.
- It allows for accurate stoichiometric calculations: Balanced equations provide the mole ratios between reactants and products, essential for determining the amounts of reactants needed and the expected yield of the product.
- It allows for quantitative analysis: Balancing is fundamental for performing quantitative analysis of reactions, including calculations involving mass, moles, and volumes.
Balancing the Equation: N₂ + H₂ → NH₃
To balance the equation N₂ + H₂ → NH₃, we need to ensure that the number of nitrogen and hydrogen atoms is equal on both sides. Let's follow a step-by-step process:
-
Count the atoms: On the reactant side, we have 2 nitrogen atoms (from N₂) and 2 hydrogen atoms (from H₂). On the product side, we have 1 nitrogen atom and 3 hydrogen atoms (from NH₃).
-
Balance nitrogen: To balance nitrogen, we need to add a coefficient of 2 in front of NH₃: N₂ + H₂ → 2NH₃. Now, we have 2 nitrogen atoms on both sides.
-
Balance hydrogen: Now, we have 6 hydrogen atoms on the product side (2NH₃ x 3H = 6H). To balance this, we need to add a coefficient of 3 in front of H₂ on the reactant side: N₂ + 3H₂ → 2NH₃. Now, we have 6 hydrogen atoms on both sides.
The balanced equation is therefore: N₂ + 3H₂ → 2NH₃
This equation now correctly represents the stoichiometry of the reaction, indicating that one mole of nitrogen gas reacts with three moles of hydrogen gas to produce two moles of ammonia gas.
The Haber-Bosch Process: Industrial Synthesis of Ammonia
The industrial synthesis of ammonia using the balanced reaction (N₂ + 3H₂ → 2NH₃) is achieved through the Haber-Bosch process. This process involves several crucial aspects:
1. Reactant Preparation
- Nitrogen Source: Nitrogen gas (N₂) is readily available from the atmosphere.
- Hydrogen Source: Hydrogen gas (H₂) is typically obtained from natural gas (methane) through steam reforming.
2. Reaction Conditions
The Haber-Bosch process employs specific reaction conditions to optimize ammonia yield:
-
High Pressure (150-300 atm): High pressure favors the forward reaction because it reduces the volume of the system (4 moles of gas on the reactant side become 2 moles on the product side). This is explained by Le Chatelier's principle.
-
High Temperature (400-500°C): Although high temperature favors the endothermic reverse reaction, a moderate temperature is used to achieve a reasonable rate of reaction. Too low a temperature would result in an impractically slow reaction rate.
-
Catalyst (Iron): An iron catalyst is used to speed up the reaction significantly. The catalyst lowers the activation energy, allowing the reaction to occur at a faster rate without significantly increasing temperature.
3. Product Separation and Recycling
- Ammonia Separation: Ammonia gas is condensed and separated from the unreacted nitrogen and hydrogen, which are then recycled back into the reactor to maximize efficiency.
4. Environmental Considerations
While the Haber-Bosch process is vital for food production, it also has significant environmental implications:
- Energy Consumption: The process is very energy-intensive, relying on vast amounts of fossil fuels, contributing to greenhouse gas emissions.
- CO₂ Emissions: The steam reforming process for hydrogen production contributes significantly to CO₂ emissions.
- Catalyst Production: Production and disposal of the iron catalyst can also generate environmental consequences.
Beyond Balancing: Understanding Reaction Kinetics and Equilibrium
Balancing the equation is just the first step in understanding the Haber-Bosch process. A thorough understanding also requires considering reaction kinetics and equilibrium.
Reaction Kinetics
Reaction kinetics studies the rate of a chemical reaction. The Haber-Bosch process is relatively slow even under optimal conditions. The catalyst plays a critical role in increasing the reaction rate by providing an alternative reaction pathway with lower activation energy.
Chemical Equilibrium
The Haber-Bosch process reaches a state of dynamic equilibrium where the rate of the forward reaction (N₂ + 3H₂ → 2NH₃) equals the rate of the reverse reaction (2NH₃ → N₂ + 3H₂). At equilibrium, the concentrations of reactants and products remain constant but are not necessarily equal. The equilibrium constant (K<sub>eq</sub>) expresses the relationship between the concentrations of reactants and products at equilibrium and is influenced by temperature and pressure.
Conclusion: The Significance of Balancing and Beyond
Balancing the equation N₂ + 3H₂ → 2NH₃ is crucial for understanding the stoichiometry of the Haber-Bosch process and for performing quantitative calculations. However, a deeper understanding of the process requires considering the reaction conditions, catalyst efficiency, reaction kinetics, and chemical equilibrium. The Haber-Bosch process highlights the interplay between theoretical chemical principles and practical industrial applications, underscoring the importance of both fundamental understanding and technological innovation in solving significant global challenges, particularly regarding food security. Ongoing research focuses on improving efficiency and reducing the environmental impact of this essential process, exploring alternative catalysts, milder reaction conditions, and renewable energy sources for hydrogen production. The future of the Haber-Bosch process lies in balancing its vital role in feeding the world with the urgent need for environmental sustainability.
Latest Posts
Latest Posts
-
Is Potassium Hydroxide Soluble In Water
Apr 24, 2025
-
Lcm Of 2 7 And 3
Apr 24, 2025
-
Elements In The Periodic Table Are Arranged According To Their
Apr 24, 2025
-
What Is The Middle Of An Atom Called
Apr 24, 2025
-
2 Or More Elements Chemically Combined
Apr 24, 2025
Related Post
Thank you for visiting our website which covers about How Do You Balance N2 H2 Nh3 . We hope the information provided has been useful to you. Feel free to contact us if you have any questions or need further assistance. See you next time and don't miss to bookmark.