How Do Mitochondria And Chloroplasts Work Together
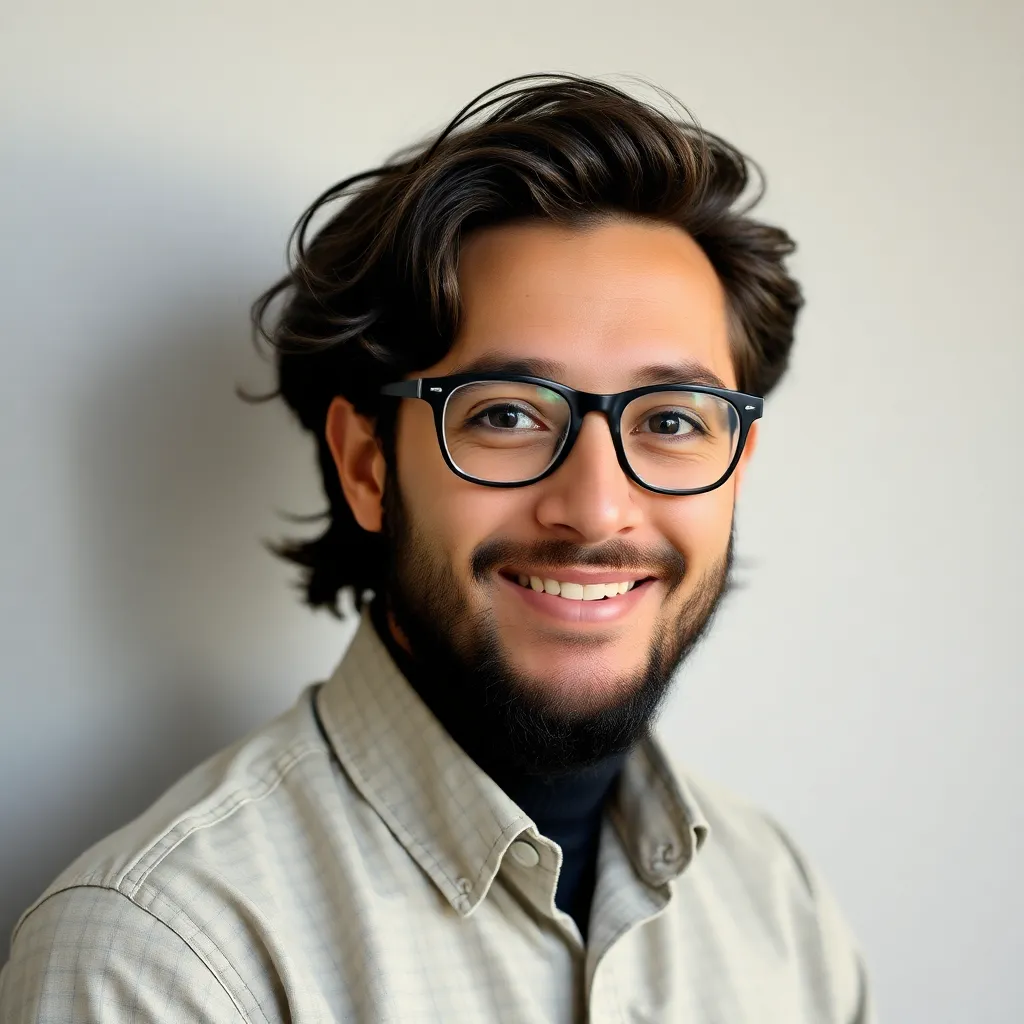
listenit
May 10, 2025 · 6 min read
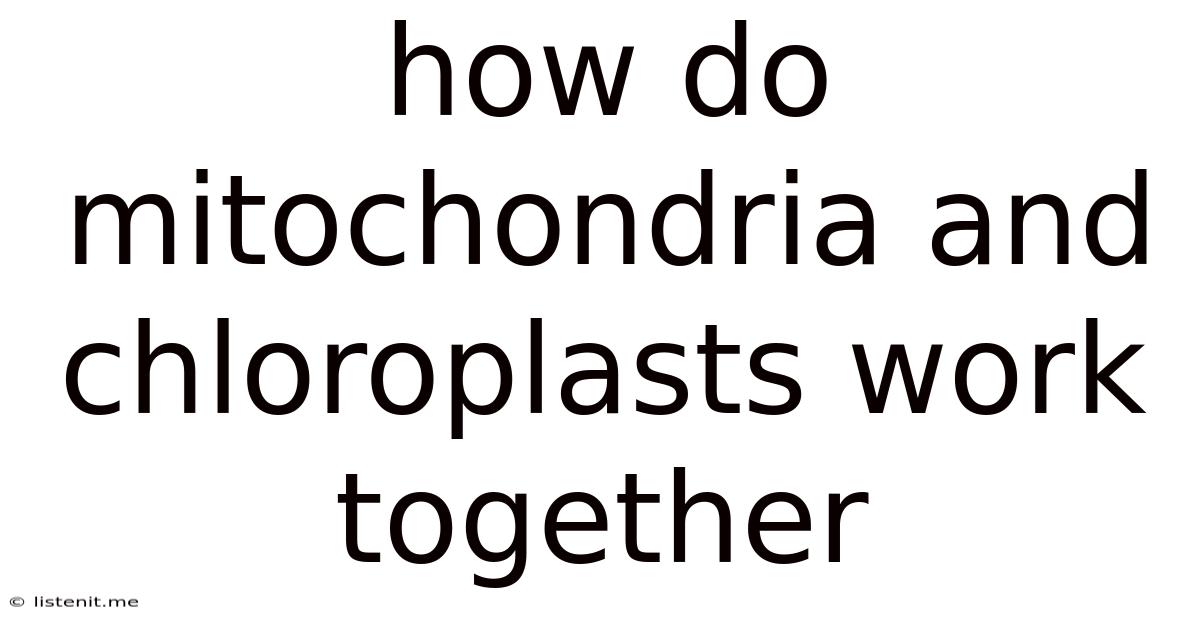
Table of Contents
How Do Mitochondria and Chloroplasts Work Together? A Symbiotic Dance of Energy
The intricate dance of life hinges on the seamless cooperation of cellular organelles. Among the most fascinating players in this cellular ballet are mitochondria and chloroplasts – two powerhouses with distinct yet intertwined roles in energy production. While mitochondria are found in almost all eukaryotic cells, powering cellular processes through respiration, chloroplasts are the exclusive domain of plant and algal cells, harnessing sunlight to synthesize sugars through photosynthesis. Despite their separate functions, these organelles share a surprising evolutionary history and exhibit a fascinating, albeit indirect, collaboration in the grand scheme of life. This article will delve into the individual functions of mitochondria and chloroplasts, exploring their evolutionary origins and how their activities, though seemingly separate, ultimately support one another in the larger ecological context.
Mitochondria: The Cellular Powerhouses
Mitochondria are often referred to as the "powerhouses" of the cell. This is because they are responsible for cellular respiration, the process of converting the chemical energy stored in glucose and other nutrients into adenosine triphosphate (ATP), the cell's primary energy currency. This complex process occurs in several stages:
1. Glycolysis: The Initial Breakdown
Glycolysis, the first stage of cellular respiration, takes place in the cytoplasm, outside the mitochondria. It involves the breakdown of glucose into pyruvate, a smaller molecule. This process generates a small amount of ATP and NADH, a high-energy electron carrier.
2. The Krebs Cycle (Citric Acid Cycle): Generating Energy Carriers
Pyruvate then enters the mitochondria, specifically the mitochondrial matrix. Here, it undergoes a series of reactions known as the Krebs cycle (also called the citric acid cycle). The Krebs cycle further breaks down pyruvate, releasing carbon dioxide as a byproduct and generating more ATP, NADH, and FADH2, another high-energy electron carrier.
3. Oxidative Phosphorylation: ATP Synthesis
The real energy powerhouse of the mitochondrion is the electron transport chain (ETC), located in the inner mitochondrial membrane. NADH and FADH2 donate their high-energy electrons to the ETC, initiating a chain of redox reactions. This electron flow drives the pumping of protons (H+) from the mitochondrial matrix to the intermembrane space, creating a proton gradient. This gradient represents stored potential energy.
Finally, the protons flow back into the matrix through ATP synthase, an enzyme that utilizes this proton motive force to synthesize ATP from ADP and inorganic phosphate (Pi). This process, called chemiosmosis, is the primary mechanism of ATP production in cellular respiration. Oxygen acts as the final electron acceptor in the ETC, forming water. Without oxygen, this process cannot continue efficiently, leading to anaerobic respiration with significantly less ATP production.
Chloroplasts: The Solar Energy Harvesters
Chloroplasts, found in plant and algal cells, are the sites of photosynthesis, the process that converts light energy into chemical energy in the form of glucose. This process can be broadly divided into two stages:
1. Light-Dependent Reactions: Capturing Light Energy
The light-dependent reactions occur in the thylakoid membranes within the chloroplast. Chlorophyll and other pigments in photosystems II and I absorb light energy. This energy excites electrons, initiating an electron transport chain similar to that in mitochondria. The electron flow generates a proton gradient across the thylakoid membrane, driving ATP synthesis via chemiosmosis. Water is split (photolysis) to replace the excited electrons, releasing oxygen as a byproduct. NADP+ is reduced to NADPH, another high-energy electron carrier.
2. Light-Independent Reactions (Calvin Cycle): Synthesizing Glucose
The light-independent reactions, also known as the Calvin cycle, take place in the stroma, the fluid-filled space surrounding the thylakoids. ATP and NADPH generated in the light-dependent reactions provide the energy and reducing power to drive the Calvin cycle. This cycle fixes atmospheric carbon dioxide (CO2) into organic molecules, ultimately producing glucose.
The Indirect Collaboration: An Ecological Perspective
While mitochondria and chloroplasts operate independently within their respective cells, their functions are intrinsically linked within the larger ecosystem. The oxygen produced by chloroplasts during photosynthesis is essential for the aerobic respiration carried out by mitochondria in both plant and animal cells. This oxygen serves as the final electron acceptor in the mitochondrial electron transport chain, driving the efficient production of ATP. Without the oxygen produced by photosynthesis, most life forms, including plants themselves, would be unable to sustain their energy needs through aerobic respiration.
Furthermore, the glucose synthesized by chloroplasts during photosynthesis serves as the primary source of energy for many organisms, including those that cannot perform photosynthesis themselves. Animals consume plants, directly or indirectly, utilizing the glucose stored in plant tissues as fuel for their own mitochondrial respiration. This continuous cycle of photosynthesis and respiration drives the flow of energy through ecosystems.
Evolutionary Connections: Endosymbiotic Theory
The striking similarities between mitochondria and chloroplasts – their double membranes, circular DNA, and independent protein synthesis – strongly suggest a shared evolutionary origin. The endosymbiotic theory proposes that mitochondria and chloroplasts were once free-living prokaryotic organisms that were engulfed by a larger host cell. This symbiotic relationship proved mutually beneficial: the host cell provided protection and nutrients, while the engulfed prokaryotes provided energy (in the case of mitochondria) or food (in the case of chloroplasts). Over millions of years, these endosymbionts evolved into the organelles we know today, integrating tightly into the eukaryotic cell's machinery.
The evolutionary relationship between mitochondria and chloroplasts further highlights their indirect interdependence. The evolution of photosynthesis in chloroplasts provided the oxygen necessary for the evolution of efficient aerobic respiration in mitochondria. This co-evolutionary journey shaped the energy dynamics of life on Earth.
Beyond the Basics: Factors Influencing Mitochondrial and Chloroplast Function
The efficiency of both mitochondria and chloroplasts can be influenced by a range of internal and external factors.
Mitochondrial Function:
- Nutrient Availability: The availability of glucose and other substrates is crucial for cellular respiration. Dietary restrictions or metabolic disorders can limit ATP production.
- Oxygen Levels: Oxygen is essential for aerobic respiration. Hypoxia (low oxygen levels) significantly impairs mitochondrial function.
- Reactive Oxygen Species (ROS): The ETC can generate ROS, which can damage mitochondrial components and DNA, leading to mitochondrial dysfunction. Antioxidant systems help mitigate this damage.
- Genetic Factors: Mutations in mitochondrial DNA (mtDNA) can cause a range of mitochondrial disorders, affecting energy production.
Chloroplast Function:
- Light Intensity: The rate of photosynthesis is directly influenced by light intensity, with optimal levels varying among species.
- CO2 Concentration: The availability of CO2 is a limiting factor for the Calvin cycle. Higher CO2 levels generally enhance photosynthesis.
- Temperature: Temperature significantly impacts enzyme activity in photosynthesis. Extreme temperatures can inhibit the process.
- Water Availability: Water is essential for photolysis, and water stress can severely reduce photosynthetic rates.
- Nutrient Deficiencies: The synthesis of chlorophyll and other photosynthetic pigments requires various nutrients. Deficiencies can impair photosynthesis.
Conclusion: A Symbiotic Partnership Shaping Life
The intricate interplay between mitochondria and chloroplasts underscores the remarkable complexity and interdependence of cellular processes. While their functions may appear distinct, their combined actions are essential for maintaining the energy balance of life on Earth. The evolutionary history and symbiotic relationship between these organelles provide a compelling example of how cooperation and interdependence have shaped the biological world, driving the evolution of diverse and complex life forms. Future research into the intricate mechanisms governing mitochondrial and chloroplast function will undoubtedly further illuminate the profound significance of their symbiotic partnership in shaping life as we know it.
Latest Posts
Latest Posts
-
Mitosis Occurs In Which Layer Of The Skin
May 10, 2025
-
Which Of The Following Problems Would Not Have A Solution
May 10, 2025
-
How Many Valence Electrons Does Group 13 Have
May 10, 2025
-
How Many Oxygen Atoms Are In Water
May 10, 2025
-
4 Is Subtracted From The Cube Of A Number
May 10, 2025
Related Post
Thank you for visiting our website which covers about How Do Mitochondria And Chloroplasts Work Together . We hope the information provided has been useful to you. Feel free to contact us if you have any questions or need further assistance. See you next time and don't miss to bookmark.