How Do Elements Heavier Than Iron Form
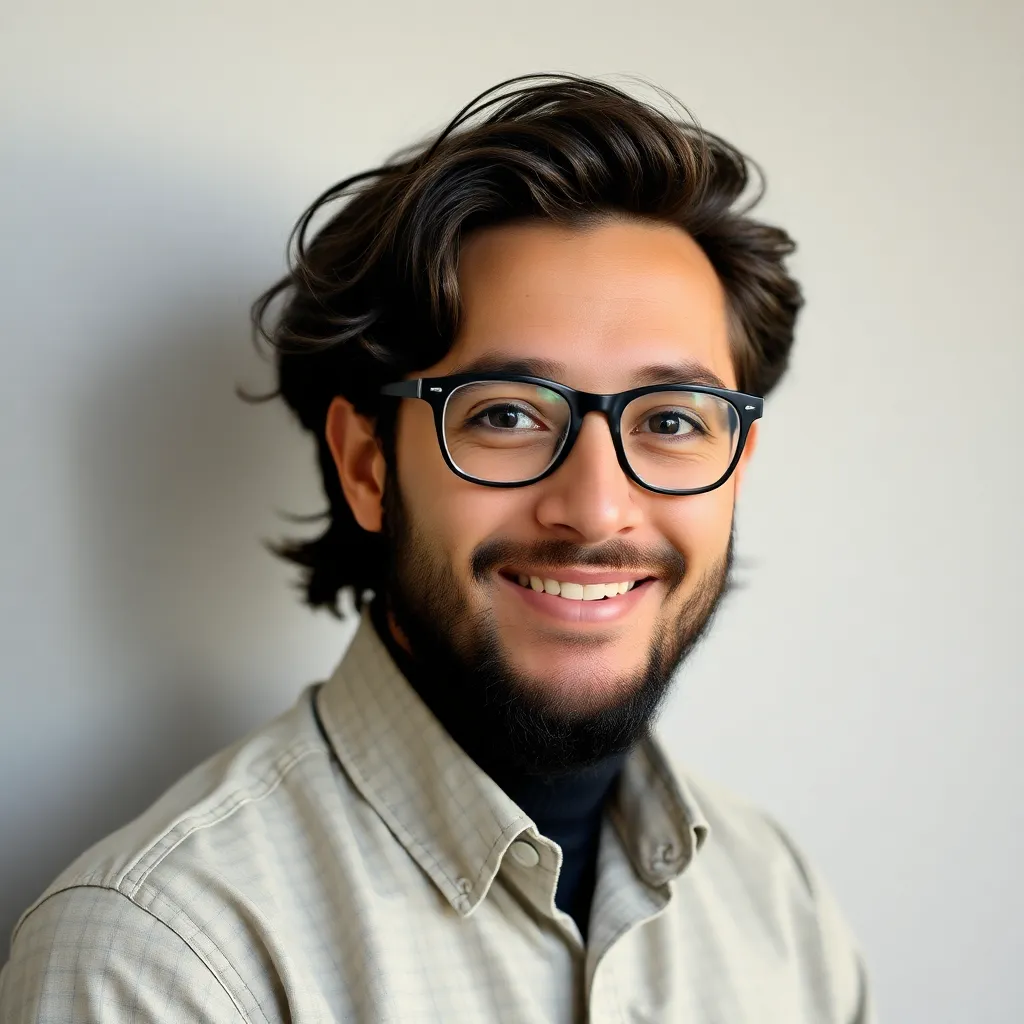
listenit
May 12, 2025 · 6 min read
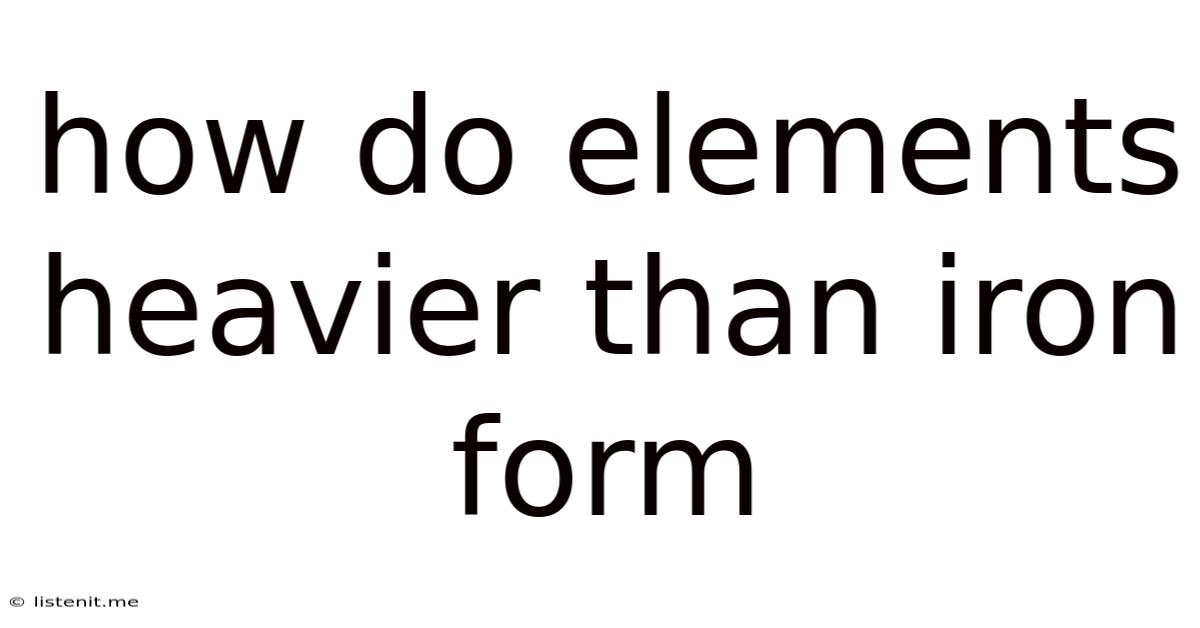
Table of Contents
How Do Elements Heavier Than Iron Form? A Deep Dive into Stellar Nucleosynthesis
The periodic table, a seemingly simple chart of elements, holds a universe of stories within its neatly arranged boxes. While lighter elements like hydrogen and helium were forged in the Big Bang, the heavier elements, including those crucial for life on Earth, have a more dramatic origin story. Understanding how elements heavier than iron are formed is a fascinating journey into the heart of stars, supernovas, and the violent processes that shape our cosmos. This article delves deep into the captivating process of stellar nucleosynthesis, specifically focusing on the creation of elements beyond iron.
The Iron Peak: A Nucleosynthetic Bottleneck
The element iron (Fe) sits at a crucial point in the nucleosynthetic process. Fusion reactions, the process by which lighter elements combine to form heavier ones, release energy up to iron. This energy release powers stars for billions of years. However, fusion reactions beyond iron require energy input, rather than releasing it. This means that forming elements heavier than iron demands a significantly different mechanism—one involving catastrophic stellar events.
Why Iron?
The stability of iron's nucleus is the key. Iron-56, the most common isotope of iron, possesses an exceptionally high binding energy per nucleon. This means that its nucleons (protons and neutrons) are incredibly tightly bound together. Further fusion to create heavier elements would require energy to overcome this strong binding, making the process energetically unfavorable under normal stellar conditions.
The Explosive Genesis: Neutron Capture Processes
The creation of elements heavier than iron primarily occurs through two neutron capture processes: the slow neutron capture process (s-process) and the rapid neutron capture process (r-process). These processes are fundamentally different in their timescale and the environment in which they occur.
The Slow Neutron Capture Process (s-process): A Gentle Build-Up
The s-process occurs in relatively calm stellar environments, specifically in asymptotic giant branch (AGB) stars. These are stars nearing the end of their lives, undergoing a period of intense helium burning in a shell surrounding the core. This helium burning releases neutrons, which are then gradually captured by existing atomic nuclei. The process is "slow" because the neutron capture rate is slower than the beta decay rate of the newly formed isotopes.
How it works:
- Neutron release: Helium burning in the AGB star's shell releases free neutrons.
- Neutron capture: Existing nuclei capture these neutrons, increasing their mass number (number of neutrons plus protons).
- Beta decay: If the resulting nucleus is unstable, it undergoes beta decay, converting a neutron into a proton and emitting an electron and an antineutrino. This increases the atomic number (number of protons).
- Iterative process: Steps 2 and 3 repeat, building up heavier and heavier elements along a stable isotopic path.
The s-process is responsible for creating about half of the elements heavier than iron found in the universe, including isotopes of elements like strontium (Sr), yttrium (Y), zirconium (Zr), and many others.
The Rapid Neutron Capture Process (r-process): A Violent Burst
In stark contrast to the s-process, the r-process takes place in extremely neutron-rich and energetic environments. The most likely sites for the r-process are kilonovae, the spectacular explosions resulting from the merger of two neutron stars or a neutron star and a black hole. These events produce a phenomenal flux of neutrons, allowing for the rapid capture of many neutrons before beta decay can occur.
How it works:
- Neutron star merger: The merger of neutron stars or a neutron star and a black hole releases an immense amount of energy and a vast number of free neutrons.
- Rapid neutron capture: Existing nuclei, often iron-group nuclei, capture neutrons at an incredibly high rate. This leads to the formation of extremely neutron-rich isotopes.
- Beta decay chain: After the neutron bombardment subsides, the neutron-rich isotopes undergo a series of beta decays, converting neutrons into protons and creating heavier elements.
- Heaviest elements: The r-process is responsible for creating the heaviest elements in the universe, including those beyond uranium (U), which are all radioactive.
The r-process is the primary source of about half of the elements heavier than iron, including many rare earth elements and the actinides. Its extreme conditions allow for the creation of elements with significantly more neutrons than typically found in stable nuclei, pushing the boundaries of the periodic table.
Other Contributing Factors: Proton Capture and Photodisintegration
While neutron capture processes are dominant, other nuclear reactions contribute to the synthesis of heavier elements.
Proton Capture (p-process): A Rare Occurrence
The p-process involves the capture of protons by nuclei, leading to the production of some proton-rich isotopes not readily formed via neutron capture. However, this process is significantly less prevalent than neutron capture and is believed to occur in the hot, explosive environments of supernovae.
Photodisintegration: Breaking Down Nuclei
Photodisintegration is the process where high-energy gamma rays break down atomic nuclei, often during the intense radiation field within a supernova. This can lead to the creation of specific isotopes and influences the abundance patterns of certain elements.
The Observational Evidence: Unveiling the Cosmic Recipe
The study of stellar nucleosynthesis isn't just theoretical. Observations from telescopes and sophisticated instruments provide crucial evidence supporting these models.
- Spectroscopy of stars: Analyzing the light from stars reveals the abundance of different elements within them, offering clues about the processes that formed them.
- Supernova observations: Studying the light curves and spectra of supernovae provides insights into the nuclear reactions occurring during these cataclysmic events.
- Kilonova observations: The detection of kilonovae and the analysis of their electromagnetic emissions confirm the role of neutron star mergers in the r-process.
- Meteorites: Meteorites offer snapshots of the early solar system, preserving the elemental abundances from the time of its formation. Analyzing the isotopic ratios in meteorites helps constrain models of nucleosynthesis.
Conclusion: A Cosmic Cookbook
The creation of elements heavier than iron is a testament to the universe's power and complexity. It's a process interwoven with the lives and deaths of stars, the violence of supernovae, and the exotic mergers of neutron stars. Understanding this intricate process not only unveils the origins of the elements that make up our world but also enhances our comprehension of the universe’s dynamic evolution. From the gentle neutron capture in AGB stars to the explosive r-process in kilonovae, each event contributes to the rich tapestry of elements that define our existence. Ongoing research continues to refine our understanding of these processes, revealing more of the universe's intricate and magnificent history. Future observations and advancements in theoretical modeling will undoubtedly unravel further mysteries in this fascinating field of stellar nucleosynthesis.
Latest Posts
Latest Posts
-
The Focal Length Of A Converging Lens Is
May 13, 2025
-
Is Zinc Carbonate Soluble In Water
May 13, 2025
-
9 Divided By Square Root Of 3
May 13, 2025
-
How To Find Nth Term Of Geometric Sequence
May 13, 2025
-
The Most Abundant Cells In The Epidermis Are
May 13, 2025
Related Post
Thank you for visiting our website which covers about How Do Elements Heavier Than Iron Form . We hope the information provided has been useful to you. Feel free to contact us if you have any questions or need further assistance. See you next time and don't miss to bookmark.