Fluid Mosaic Model Why Is It Called
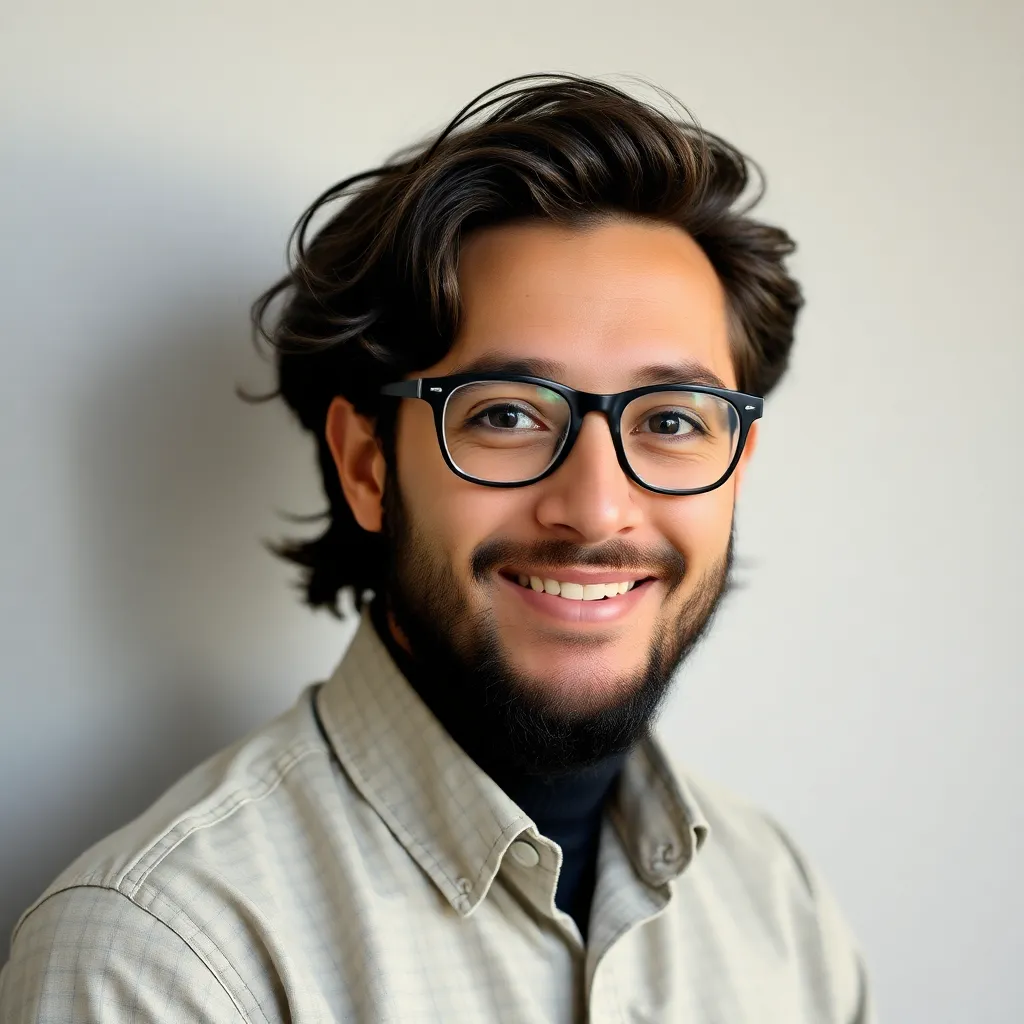
listenit
Apr 26, 2025 · 6 min read

Table of Contents
The Fluid Mosaic Model: Why This Name? A Deep Dive into Cell Membrane Structure
The cell membrane, that incredibly thin yet incredibly important barrier surrounding every living cell, is far more complex than its simple description might suggest. Understanding its structure is fundamental to grasping how cells function, interact, and maintain life itself. The prevailing model used to describe this structure is the fluid mosaic model. But why is it called that? Let's delve deep into the intricacies of this model, exploring the "fluid" and the "mosaic" aspects to understand the name's perfect fit.
The "Fluid" Aspect: A Dynamic Structure in Constant Motion
The term "fluid" in the fluid mosaic model highlights the dynamic and flexible nature of the cell membrane. It's not a rigid, static structure; instead, it's a constantly shifting, flowing landscape of molecules. This fluidity arises primarily from the nature of its major components: phospholipids.
Phospholipid Bilayer: The Foundation of Fluidity
The cell membrane is primarily composed of a phospholipid bilayer. Each phospholipid molecule has a hydrophilic (water-loving) head and two hydrophobic (water-fearing) tails. This amphipathic nature is crucial to the membrane's structure. In an aqueous environment, the phospholipids spontaneously arrange themselves into a bilayer, with the hydrophilic heads facing the watery environments inside and outside the cell, and the hydrophobic tails tucked away in the interior, shielded from water.
This bilayer, however, isn't static. The phospholipids are constantly moving, exhibiting lateral diffusion, meaning they move sideways within their own monolayer. They can also rotate around their axes and, less frequently, undergo flip-flop, moving from one monolayer to the other. This constant movement contributes significantly to the membrane's fluidity.
Factors Influencing Membrane Fluidity
Several factors influence the fluidity of the cell membrane:
-
Temperature: Higher temperatures increase the kinetic energy of the phospholipids, making them more fluid. Conversely, lower temperatures decrease fluidity, potentially leading to a more rigid, gel-like state.
-
Fatty Acid Saturation: Phospholipids with unsaturated fatty acids (containing double bonds) have kinks in their tails, preventing them from packing tightly together. This leads to increased membrane fluidity. Saturated fatty acids, lacking double bonds, pack more closely, resulting in reduced fluidity.
-
Cholesterol: Cholesterol, a crucial component of animal cell membranes, acts as a fluidity buffer. At high temperatures, it restricts phospholipid movement, decreasing fluidity. At low temperatures, it prevents phospholipids from packing too tightly, maintaining fluidity.
The fluidity of the membrane is essential for a multitude of cellular processes. It allows for:
- Membrane protein mobility: Proteins embedded in the membrane can move laterally, facilitating interactions and functions.
- Cell growth and division: The fluidity allows for membrane expansion and reshaping during cell growth and division.
- Endocytosis and exocytosis: The fluidity enables the membrane to engulf materials (endocytosis) or release materials (exocytosis).
- Signal transduction: The movement of membrane receptors facilitates signal transmission across the membrane.
The "Mosaic" Aspect: A Diverse Collection of Embedded Molecules
The "mosaic" part of the fluid mosaic model refers to the diverse array of proteins and other molecules embedded within the phospholipid bilayer. These molecules aren't uniformly distributed; rather, they form a complex pattern resembling a mosaic. This heterogeneity is crucial for the membrane's diverse functions.
Membrane Proteins: The Functional Diversity
Membrane proteins are categorized into two major groups based on their association with the bilayer:
-
Integral proteins: These proteins are embedded within the bilayer, often spanning the entire width (transmembrane proteins). They typically have hydrophobic regions interacting with the lipid tails and hydrophilic regions exposed to the aqueous environments. Integral proteins perform various functions, including:
- Transport: Facilitating the movement of ions and molecules across the membrane.
- Receptors: Binding to signaling molecules and initiating intracellular responses.
- Enzymes: Catalyzing biochemical reactions at the membrane surface.
- Cell adhesion: Connecting cells to each other or to the extracellular matrix.
-
Peripheral proteins: These proteins are loosely associated with the membrane surface, often interacting with the hydrophilic heads of phospholipids or integral proteins. They typically play roles in:
- Signal transduction: Relaying signals from membrane receptors to intracellular targets.
- Cytoskeletal support: Connecting the membrane to the cytoskeleton.
Other Membrane Components: Completing the Mosaic
Beyond phospholipids and proteins, the cell membrane also contains other crucial components that contribute to its mosaic nature:
-
Carbohydrates: Glycolipids and glycoproteins (carbohydrates attached to lipids or proteins) are found on the outer surface of the membrane. They play roles in cell recognition, cell signaling, and protection.
-
Cholesterol (in animal cells): As mentioned earlier, cholesterol influences membrane fluidity and stability.
This intricate arrangement of diverse molecules, each with its specific location and function, creates the "mosaic" aspect of the model. The non-uniform distribution of these components is crucial for the membrane's ability to carry out its diverse tasks efficiently.
The Significance of the Fluid Mosaic Model
The fluid mosaic model revolutionized our understanding of cell membranes. Before its proposal, the membrane was often envisioned as a static, rigid structure. The model's emphasis on fluidity and molecular diversity provided a far more accurate and comprehensive picture. It elegantly explains how:
- Selective permeability: The membrane's structure allows it to regulate the passage of substances, selectively letting some in while keeping others out. This is essential for maintaining cellular homeostasis.
- Signal transduction: The movement of membrane receptors and other proteins facilitates communication between cells and their environment.
- Cell-cell interactions: The mosaic nature of the membrane allows for specific interactions between cells, crucial for tissue formation and function.
- Membrane trafficking: The fluidity and dynamic nature of the membrane are essential for processes like endocytosis and exocytosis, which involve membrane fusion and fission.
Beyond the Basic Model: Ongoing Refinements
While the fluid mosaic model provides a robust framework for understanding cell membranes, it's important to acknowledge that it's a simplification of a highly complex system. Research continues to reveal further intricacies and nuances:
-
Membrane microdomains: The membrane isn't entirely homogenous; specific regions, such as lipid rafts, exhibit distinct lipid and protein compositions and play specialized roles.
-
Membrane curvature: The membrane's curvature isn't uniform and plays a significant role in various cellular processes, like vesicle formation.
-
Dynamic interactions: The interactions between membrane components are not static but constantly evolving, influenced by cellular signals and environmental factors.
Conclusion: A Fitting Name for a Complex Structure
The name "fluid mosaic model" aptly captures the essence of the cell membrane's structure and function. The "fluid" aspect emphasizes the dynamic and flexible nature of the phospholipid bilayer, while the "mosaic" aspect highlights the diverse collection of proteins and other molecules embedded within it. This model, while continually refined by ongoing research, remains a cornerstone of cell biology, offering a powerful and elegant explanation for the remarkable capabilities of this essential cellular component. Understanding the fluid mosaic model is fundamental to appreciating the complexity and beauty of life at the cellular level. The ongoing research continues to unveil further intricacies, solidifying its importance in the field of cell biology and beyond. The model’s adaptability to new findings underscores its enduring relevance and power in explaining the multifaceted functions of this crucial biological structure.
Latest Posts
Latest Posts
-
Common Denominator Of 3 And 8
Apr 27, 2025
-
What Is The Least Common Multiple Of 9 And 10
Apr 27, 2025
-
What Is The Gcf Of 12 30
Apr 27, 2025
-
Whats 70 Degrees Fahrenheit In Celsius
Apr 27, 2025
-
Does Constant Speed Mean Constant Velocity
Apr 27, 2025
Related Post
Thank you for visiting our website which covers about Fluid Mosaic Model Why Is It Called . We hope the information provided has been useful to you. Feel free to contact us if you have any questions or need further assistance. See you next time and don't miss to bookmark.